Read Part 1 on defining distributed maritime operations.
Read Part 2 on anti-ship firepower and U.S. shortfalls.
By Dmitry Filipoff
Massed Fires – A Core Tactic of Distributed Warfighting
A core tactic that operationalizes the concept of concentrating effects without concentrating platforms is combining the missile firepower of widely distributed forces. As various platforms launch weapons, their contributing fires combine to grow an overall aggregate salvo that is directed against a shared target. As commanders look to defeat and defend fleets, their decision-making will be strongly influenced by shaping the potential of these massed fires. These methods of massing missile firepower can form a centerpiece of fleet combat tactics in the modern era.
Because even one missile hit can be enough to put a ship out of action, modern high-end warships tend to emphasize powerful air defenses, which can include anti-air weapons, point defenses, electronic warfare, decoys, and other means. These many defenses significantly drive up the volume of fire needed to overwhelm warships and score hits. This makes the ability to mass anti-ship fires from distributed forces a valuable method for mustering enough volume of fire to threaten naval formations.
The adage of “firing effectively first” has sometimes been based in winning the scouting competition that precedes the launching of fires.1 But one can certainly find the adversary first while not having enough available firepower to overwhelm their defenses. It is possible for opposing naval formations to effectively target one another, but are forced to hold fire until more additional launch platforms are made available to add enough contributing fires. A critical component of firing effectively first is being the first to launch enough volume of fire to overwhelm warship defenses.
The current inventory of only eight Harpoons or Naval Strike Missiles on many U.S. surface combatants is hardly enough to be a credible threat to many modern warships. However, if warships carrying only a few missiles apiece can be credibly augmented by more anti-ship fires delivered by bombers, submarines, and other platforms, then the individual warship presents a much larger and amorphous threat. The individual warship features as part of the greater whole that is the distributed force, because a small salvo launched by one platform could very well mean that more salvos from more platforms are on the way. Warships fielding small loads of missiles cannot be discounted or viewed in isolation from the larger force, which magnifies the threat posed by even lightly-armed combatants. Therefore the ability to mass fires considerably broadens the extent of force distribution in the eyes of the adversary.
Contributing Fires and Aggregation Potential
Massed fires can combine multiple different types of missiles, which can be done for the sake of presenting more distributed threats, preserving certain types of weapon inventory, or making due with whatever firepower is available. However, combining fires from a variety of platforms fielding a variety of weapons will pose challenges. Commanders must understand what characteristics dictate the options for how massed fires can take shape, and how these options affect the distribution and risk profile of their forces.
Each individual act of contributing fires to an aggregating salvo can have a narrow window of opportunity measured in only the tens of seconds.2 Launching too late or too early will amount to launching an entirely separate salvo, and risk having missiles suffer defeat in detail while forsaking the advantages of combining fires. To effectively overwhelm multiple layers of air defenses, the missiles of an aggregated salvo have to tightly overlap the target within a similar timeframe, such as within the critical two-minute timeframe that subsonic sea-skimming missiles are visible to a target warship after they break over the horizon. Coordinated timing is central to concentrating firepower.
Regardless of the range or speed of the types of missiles, they will combine over a target if their time to reach the target is similar. One salvo does not need to physically merge with another salvo on the way to the target so long as their time to reach the target overlaps. However, the firing sequence will be affected by how different missiles have different ranges, and how quickly their speed allows them to travel those ranges. The desired timing of strikes affects the sequencing and availability of distributed launches.
Although contributing fires must overlap the target at a similar time, the fires may not all be launched at a similar time. If the U.S. Navy wanted to fire each type of its anti-ship weapons at the same time and have them strike at the same time, then all launch platforms would have to be roughly within the small 80-mile range of the Harpoon missile. The SM-6 launch platform would be a few dozen miles further out because of the weapon’s greater speed. More realistically, taking advantage of a variety of weapon ranges means distributed forces will be at different distances from the same target, and will have to sequence their launches to combine fires. A core task of assembling massed fires is organizing these firing sequences, and understanding the tactical implications of their design.
A critical factor is how long it takes a type of missile to fly to the limit of its range. Assuming the missile can be targeted out to this distance, the maximum flight time creates thresholds and ceilings for how much opportunity the missile has to combine with other fires. Missiles with longer flight times or longer ranges have more aggregation potential and offer more opportunity to combine with other fires. But if missiles have to be fired from a variety of ranges, then missiles with shorter times-to-target will have to wait on missiles with longer times to combine with them.
The maximum flight time of LRASM is estimated here at slightly less than 40 minutes. 3 If LRASM fires are to combine with a separate salvo, then that salvo must also be 40 minutes away or less from striking the target. Once these two factors come close to overlapping – the time-to-target of the waiting contributing fires and the time-to-target of the traveling aggregated salvo – those contributing fires will then have tens of seconds of opportunity to launch and effectively combine with the salvo. The figures below show roughly how long it takes U.S. anti-ship missiles to travel their maximum ranges at their maximum speeds, highlighting a critical factor of aggregation potential (Figures 1 and 2).

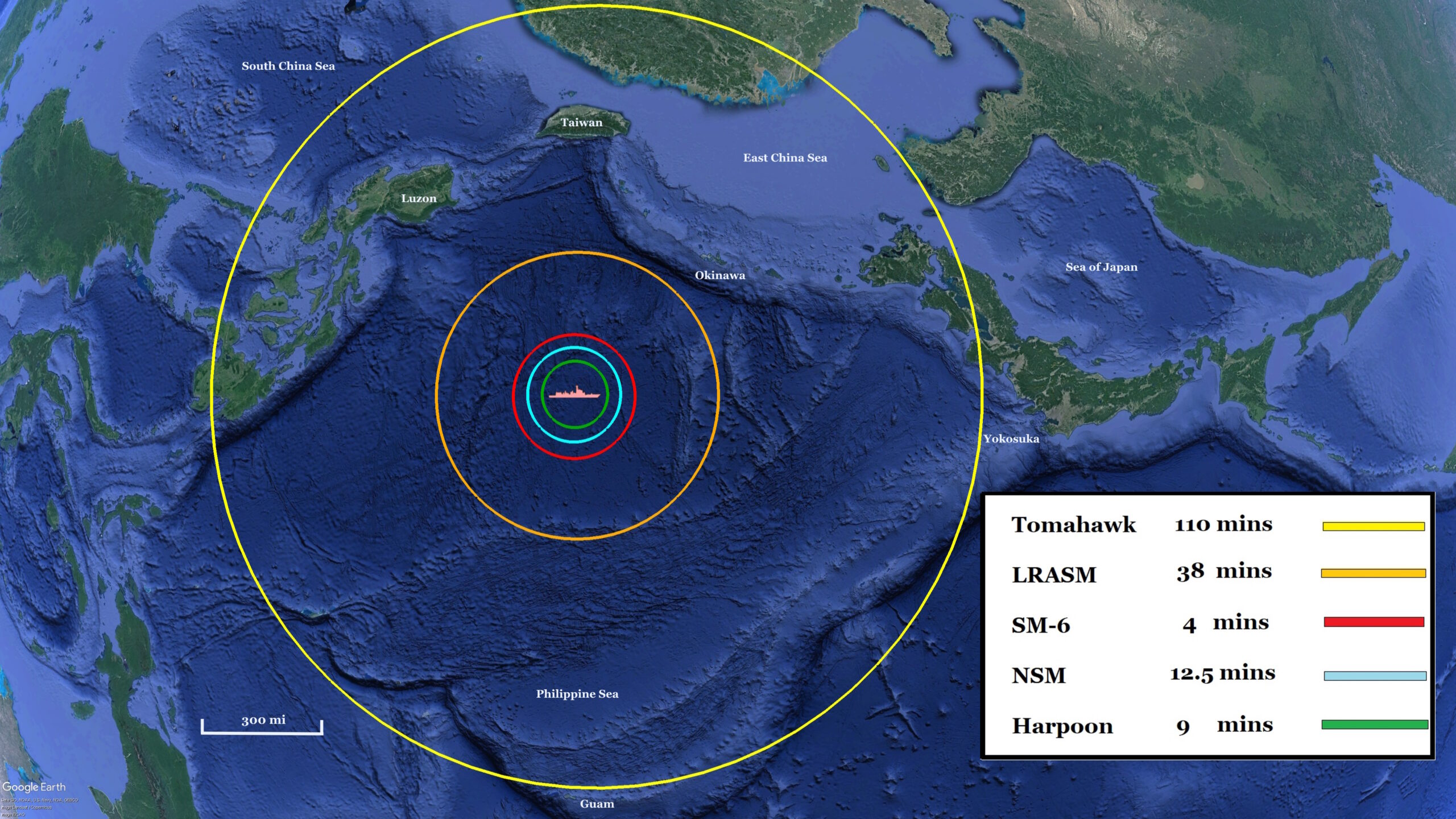
If missiles of similar speeds are to be combined to grow the volume of fire, then the weapon with the shorter range must wait for the longer-ranged weapon to close enough distance to make combination possible. When range overlaps, the time-to-target will also overlap for missiles of similar speed. Once the longer-ranged weapon aligns with the range of the shorter-ranged weapon, then the latter can be launched to combine fires. If a Harpoon salvo is to combine with a Tomahawk salvo, then the Harpoon launchers must wait for the Tomahawk salvo to be 80 miles or less away from the target to be able to combine with the salvo.
Assuming launch platforms will try to make the most of the range of their weapons, platforms firing Tomahawk will often fire first and platforms launching any other U.S. anti-ship missile will be firing much later in the firing sequence. By necessity those other platforms will have to be much closer to the target than those firing Tomahawk. They could have to wait as long as an hour or more for a Tomahawk salvo to get close enough for them to combine fires.
Combining weapons of widely differing speeds can require limiting tactical opportunities to create a viable firing sequence and achieve a larger volume of fire. The fastest weapons will often have to be fired last in sequence so they can catch up to slower weapons within the narrow timeframe of overlapping the target (Figure 3). The platforms with the fastest weapons will often have to wait the longest to fire, even though they may face the greatest pressures and opportunities to fire first. The potential of capitalizing on a faster weapon’s ability to strike a target earlier can be constrained by the need to combine with slower weapons to achieve enough volume of fire. This constraint stems from the relatively rare nature of the fastest weapons and how subsonic missiles are more common. Otherwise, firing salvos wholly composed of the most high-end and faster missiles can be especially expensive, depleting, and a less distributed form of massing firepower.
Consider how when firing an SM-6 missile in a standalone attack, a target can have as little as four or less minutes of potential warning against the incoming strike. But when SM-6 is a part of contributing fires, the missile’s launch platform will be forced to wait until the aggregated salvo is around four or less minutes away from striking before the SM-6 can be fired.
Figure 3. Click to expand. Three warships launch contributing fires of equal speed that surpass a fourth warship (USS Arleigh Burke). The fourth warship is still able to combine fires by using missiles of higher speed. (Author graphic via Nebulous Fleet Command)
But faster weapons offer many advantages, such as how they can help an aggregating salvo recover from failing or failed strikes. They can be quick enough to be inserted into an active firing sequence, giving commanders flexible options to augment the salvo as it is unfolding. If contributing fires are destroyed on the way to the target, high-speed weapons can be fired to recover lost volume and bolster the salvo into overwhelming dimensions (Figure 4). If a salvo is defeated by defenses, but those defenses were heavily depleted of anti-air weapons in the process, then high-speed weapons can quickly seize the opportunity to finish the target. Faster weapons can also spare commanders from the lengthier process of organizing fires from slower weapons when needed.
Figure 4. Click to expand. Faster missiles are used to recover lost volume of fire after a set of slower contributing fires suffer attrition. (Author graphic via Nebulous Fleet Command)
Yet in the context of a massed firing sequence, even if a platform fields the fastest missile, it could be the last to fire. It may have to wait the longest even though it could hit the earliest. The longer a platform has to wait for its turn in the firing sequence, the more opportunity the adversary will have to preemptively attack the archer before it can contribute its fires. As commanders organize mass fires, they must be wary of the predictability of their firing sequences and the risk of suffering interruptive strikes.
The Risks of Predictability and Interruptive Strikes
The way a distributed posture is presented to an adversary will flex and evolve during the course of a mass firing sequence. As an aggregating salvo closes in on a target, the options for growing the volume of fire will narrow, and the remaining distribution of potential launch platforms becomes increasingly concentrated. These dynamics simplify some of the adversary’s targeting challenges, where a force will strive for broad-area awareness partly to understand how an adversary’s massed fires are coming together and pinpoint opportunities to disrupt the firing sequence as it is unfolding.
The staggered nature of building an aggregated salvo from sequenced fires increases the risk to friendly platforms whose contributing fires come later in the firing sequence. If an adversary discovers that standoff fires are being launched against them from distant forces, they may view closer forces as pressing targets demanding immediate strikes. Those closer forces are potential candidates for contributing to the volume of the incoming salvo. They could be archers waiting their turn. By targeting these forces before the salvo gets close enough to be combined with, a defender can preemptively destroy platforms to restrict the growth of the salvo and kill targets with fuller magazines (Figure 5).
Figure 5. Click to expand. Sensing a mass firing sequence, an adversary launches high-speed missiles at a pair of warships it believes will soon add contributing fires. (Author graphic via Nebulous Fleet Command)
When a firing sequence is initiated and an aggregated salvo is born, the burden of destroying archers before they fire arrows considerably intensifies. But those distributed archers must realize that a friendly salvo fired by someone else can make them prime targets of opportunity. If a platform has to wait an hour or more to combine fires with a Tomahawk salvo, then that can offer plenty of time for them to be preemptively attacked by an adversary. The earlier a platform can launch in the firing sequence, the more it reduces its attractiveness for preemptive strikes during the course of assembling massed fires.
The process of assembling massed fires will take on a much more predictable pattern when most of a military’s anti-ship missiles have similar speeds, such as the U.S. military’s mostly subsonic arsenal. In this case an aggregated salvo can take the predictable pattern of gradually building in volume as it closes the range to the target. The outermost platforms initiate the strike by firing the longest-ranged weapons, then platforms closer to the target and with shorter-ranged missiles contribute their fires in turn. As the aggregated salvo closes the distance, each platform that becomes further away from the target than the salvo can be ruled out as a candidate for adding more contributing fires. The potential scope of remaining fires and launch platforms predictably shrinks as the aggregated salvo gets closer to the target. As the salvo closes the distance, the resulting distribution of potential contributors becomes tighter and more concentrated, making clearer to the adversary which archers may remain (Figure 6).
Figure 6. Click to expand. A mass firing sequence takes on a predictable pattern of aggregation by using missiles of similar speed. (Author graphic via Nebulous Fleet Command)
This predictability can be mitigated through several measures, including by combining fires with weapons featuring widely different speeds. Platforms with faster weapons can remain a candidate for contributing fires even if they are further away from the target than the aggregated salvo, which helps preserve force distribution as the salvo closes in (Figure 7). An adversary that sees an incoming salvo of Tomahawks 100 miles away can rule out that any platform well beyond that range cannot add further Tomahawks to that salvo. But warships 150 miles away can still pose a threat by launching SM-6s that are fast enough to catch up to the Tomahawks and combine over the target in the final minutes.
Figure 7. Click to expand. A mass firing sequence takes on a less predictable form of aggregation by combining missiles of mixed speeds. (Author graphic via Nebulous Fleet Command)
In a similar vein, Chinese forces firing subsonic anti-ship weapons can still have ballistic and hypersonic missiles combine with their fires, despite those faster weapons being launched from positions that are potentially hundreds of miles behind the platforms firing the subsonic weapons. Weapons with a combination of extremely long range and high speed can be on call to rapidly combine with a large variety of other salvos on a theater-wide scale. Forces fielding weapons with a variety of speeds therefore present more complex forms of distribution that make it more difficult to predict how their contributing fires can come together.
Waypointing is a critical tactic that can make aggregation less predictable and complicate an adversary’s options for preemptively striking waiting archers. Weapons with both long range and long flight times can allow commanders to program waypoints into flight paths to artificially increase the time-to-target and therefore lengthen the opportunity to combine fires. Waypointing can allow platforms closer to the target to launch their contributing fires earlier than if they had simply waited for their time-to-target to overlap with the traveling aggregated salvo.
Consider a warship that is waiting to contribute fires to a salvo that is 30 minutes further away from striking a target than the warship’s own fires. Waypointing can allow that warship to fire immediately and make up the time difference through nonlinear flight paths (Figure 8). This tactic of waypointing contributing fires can allow warships to deprive adversaries of the opportunity to destroy archers before they fire arrows, even if those archers can have a shorter time-to-target than the salvos they are aggregating with.
Figure 8. Click to expand. A pair of warships much closer to the target than distant platforms uses waypointing to launch early in the firing sequence while still aligning the time-to-target with the other contributing fires. (Author graphic via Nebulous Fleet Command)
When contributing fires consist of weapons with similar speeds, the methods of waypointing and in-flight retargeting can allow those salvos to not only combine over the target, but to also merge together on the way to the target. By selectively merging contributing fires and creating more distinct masses earlier in the firing sequence, an attacker can manipulate an adversary’s perceptions and lure defensive airpower toward certain directions. Merging contributing fires can make an adversary falsely perceive that a given formation fired a larger salvo than is actually the case, which can create illusions of greater force concentration and magazine depletion (Figure 9). An adversary may believe a formation is more heavily armed and concentrated than previously believed and redirect more attention toward it. Or the adversary could believe the formation has diminished its value as a potential target by assuming it depleted much of its offensive firepower, and redirect attention away from it.
Figure 9. Click to expand. Two naval formations of several warships use waypointing to give the impression that a large standalone salvo was fired from the vicinity of a single warship (USS Mustin). (Author graphic via Nebulous Fleet Command)
By offering the ability to artificially increase the time-to-target, waypointing allows a force to make its firing sequences much more unpredictable in how they unfold. The path a waypointed salvo can take to the target is not linear, making it unclear to the adversary when exactly the salvo may arrive, what it is targeting, and what other contributing fires it may combine with. A sequence of waypointed fires may not predictably grow an aggregated salvo from the outside in. Rather, each platform uses waypointing to align its contributing fires with the time-to-target of other salvos that are being fired from a variety of ranges and are taking a variety of paths to the target. Through waypointing, the order of the firing sequence is no longer purely defined by who is farther or closer to a target, complicating the adversary’s ability to set priorities for interruptive strikes. This method is potentially one of waypointing’s most powerful force multipliers for enhancing distribution.
Figure 10. Click to expand. Distributed forces launch a mass firing sequence that consists entirely of waypointed salvos. (Author graphic via Nebulous Fleet Command)
Creative methods of assembling massed fires are not only useful for producing overwhelming firepower, but for manipulating the adversary’s interpretations of massed fires for tactical effect. In line with the fundamental tenets of distributed warfighting, missile waypointing is a valuable means of challenging an adversary through complex threat presentations.
Distributing Volume of Fire Across Time
At what point in the firing sequence will the aggregated salvo take on enough volume to be overwhelming? As various contributing fires are launched during the course of massed fires, tactical advantage and disadvantage will come into play depending on when exactly the salvo reaches overwhelming volume on its way to the target. Preserving distribution is not only a matter of managing the physical locations of platforms and contributing fires, it is also a matter of distributing launches across points in time within a firing sequence. Well-distributed launch timing can allow a volume of fire to grow robustly yet unpredictably. Understanding the distribution of launches across time is central toward knowing how to disrupt a massed firing sequence through interruptive strikes and to secure tactical advantage.
A backloaded firing sequence depends on contributing fires to push the aggregate salvo into overwhelming dimensions near the end of the firing sequence. If an aggregated salvo does not reach overwhelming volume until the firing sequence is almost over, then the attack is more fragile and easily disrupted by attacking the contributing fires and waiting archers. A long-range Tomahawk salvo that heavily depends on combining with Harpoon salvos launched by an air wing would take the form of a backloaded firing sequence.
A frontloaded scheme achieves overwhelming volume of fire early in the firing sequence. A large amount of contributing fires are launched early on, but the salvo receives few if any contributing fires for the rest of the firing sequence. The adversary can focus more of their attention and command and control on managing defenses, because a frontloaded firing sequence can spare the adversary the pressure of having to rapidly initiate their own firing sequence in pursuit of interruptive strikes. Multiple warships firing large Tomahawk salvos in tandem and from distant standoff ranges would take the form of a frontloaded firing sequence.
These two schemes of firing sequences – frontloaded and backloaded – are disadvantaged forms of concentration with respect to timing. Various drawbacks are incurred by concentrating the growth of the volume of fire toward the frontend or backend of a firing sequence. If an adversary confronts a distributed force that repeatedly uses concentrated firing sequences, then distribution is diminished and massed fires become more predictable.
A well-distributed firing sequence makes the growth of the volume of fire less predictable and combines the advantages of frontloaded and backloaded schemes. By achieving high volume of fire early in the sequence like a frontloaded scheme, more contributing fires can be added later to increase the margin of overmatch and ensure the salvo can remain overwhelming. There will be more opportunity for new launches to join the active firing sequence, especially to recover volume of fire if it is lost to attrition or if friendly platforms are preemptively destroyed before they can contribute fires.
By also featuring a meaningful number of launches later in the firing sequence, distributed launch timing can make an adversary believe that both offensive and defensive actions are necessary to restrict the growth of the salvo. They may believe they must preemptively attack waiting archers to interrupt the firing sequence and inhibit the growing volume of fire. Adversaries would feel pressed to defend against missiles while also interrupting an active firing sequence through striking waiting platforms, stretching their decision-making across both offensive and defensive efforts.
A well-distributed firing sequence may be more logistically intensive, where a force would expend enough munitions to achieve overwhelming volume of fire early in the sequence, and still have plenty more launches occur later. This sort of firing pattern is more depleting, but it achieves the critical aim of reducing dependence on launches later in the firing sequence while still leveraging them to enhance distribution and further grow the volume of fire. Ideally an aggregated salvo has enough volume of fire to not only remain overwhelming against enemy defenses, but to also remain overwhelming when multiple friendly archers have been destroyed before they could contribute their planned fires. Launching enough volume of fire to withstand disrupted firing sequences will add to the extreme expense and potential for overkill that characterizes this form of warfare.
The pressure to interrupt an active firing sequence can force commanders to expend more of their fastest and most high-end weapons in interruptive strikes. These weapons can have low enough flight times that they can be fired after an adversary initiates massed fires and still reach targets in time to disrupt the firing sequence. Subsonic salvos by comparison will have far less potential for interruptive strikes. There may be significant opportunity to disrupt the massed fires of the U.S. Navy when its principal land-attack and anti-ship cruise missile will be a weapon that can take almost two hours to travel to the limits of its range, and when China fields anti-ship ballistic missiles of similar range that can reach targets within 15 minutes.5
The distribution of maximum flight times across U.S. anti-ship missiles will make for a more backloaded firing sequence when more weapons have to combine with Tomahawk fires (Figure 11). If Tomahawk is to be fired from near the limits of its range yet still combine with other types of anti-ship weapons, then the launch platforms firing those other weapons will have to wait around an hour before they reach their turn in the firing sequence. A shorter overall firing sequence can be achieved by foregoing Tomahawks and using the other U.S. anti-ship weapons, but those weapons require much denser platform concentration to mass enough fires, especially for air wings. The U.S. can accomplish a well-distributed firing sequence mainly by having enough Tomahawk shooters throughout the battlespace and at widely different ranges from targets, while also leveraging the missile’s potent waypointing and retargeting capabilities. The figures below illustrate different forms of distribution and concentration across firing sequence timelines (Figures 12-14).
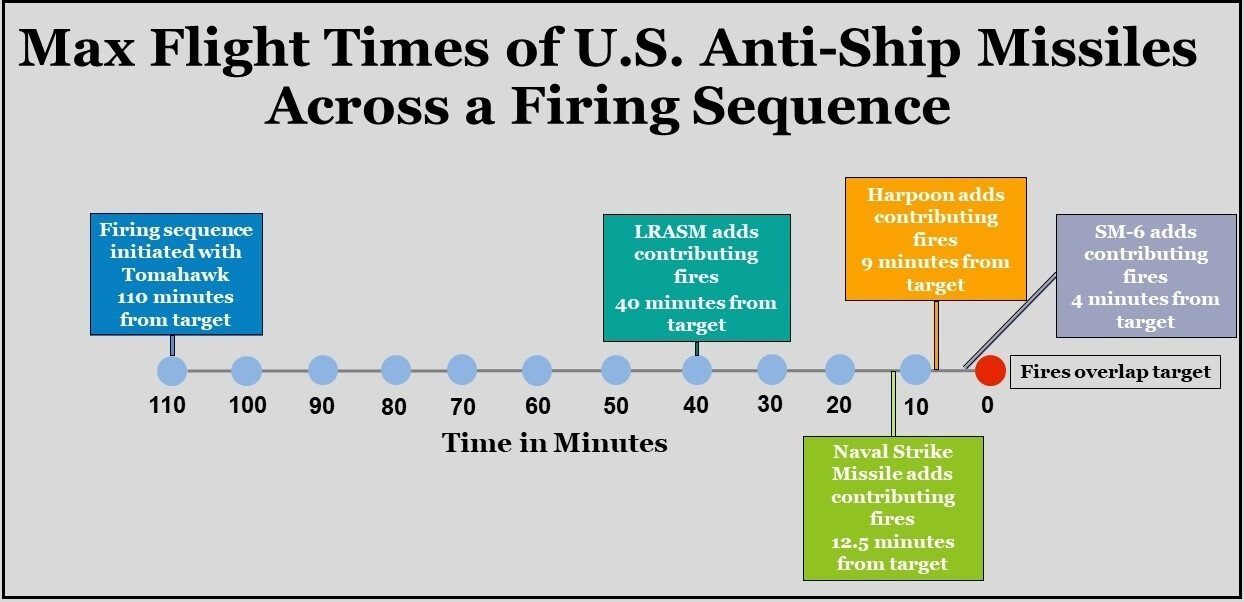
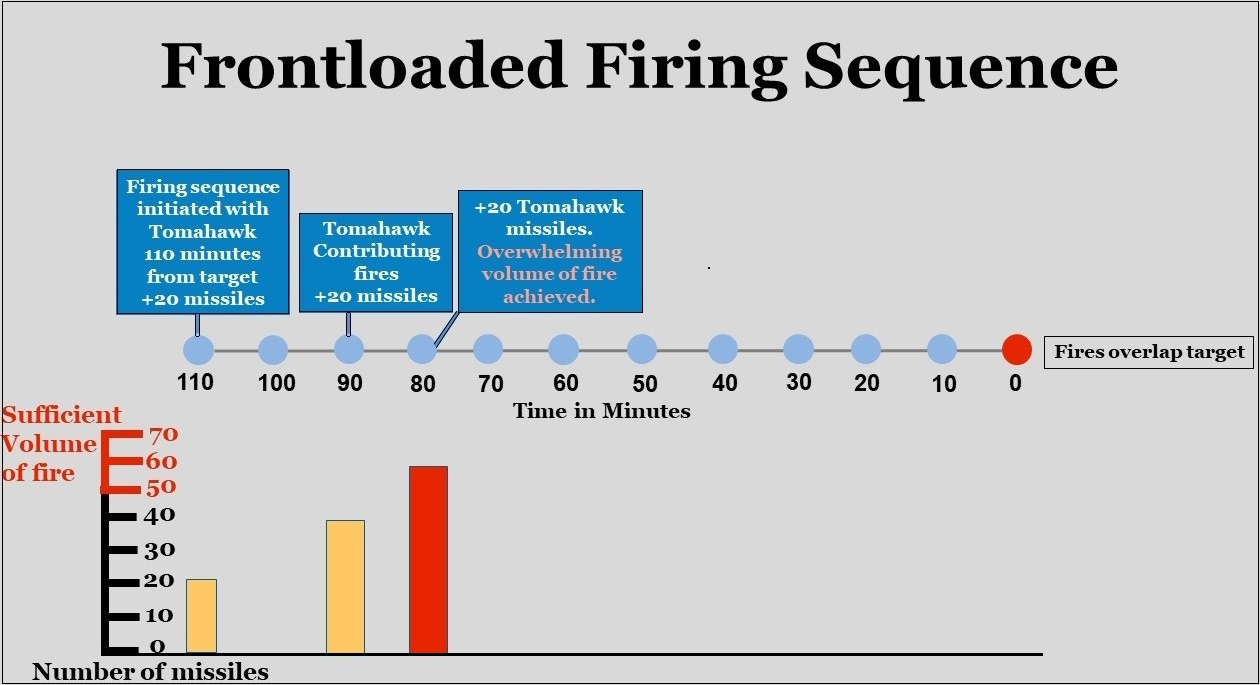
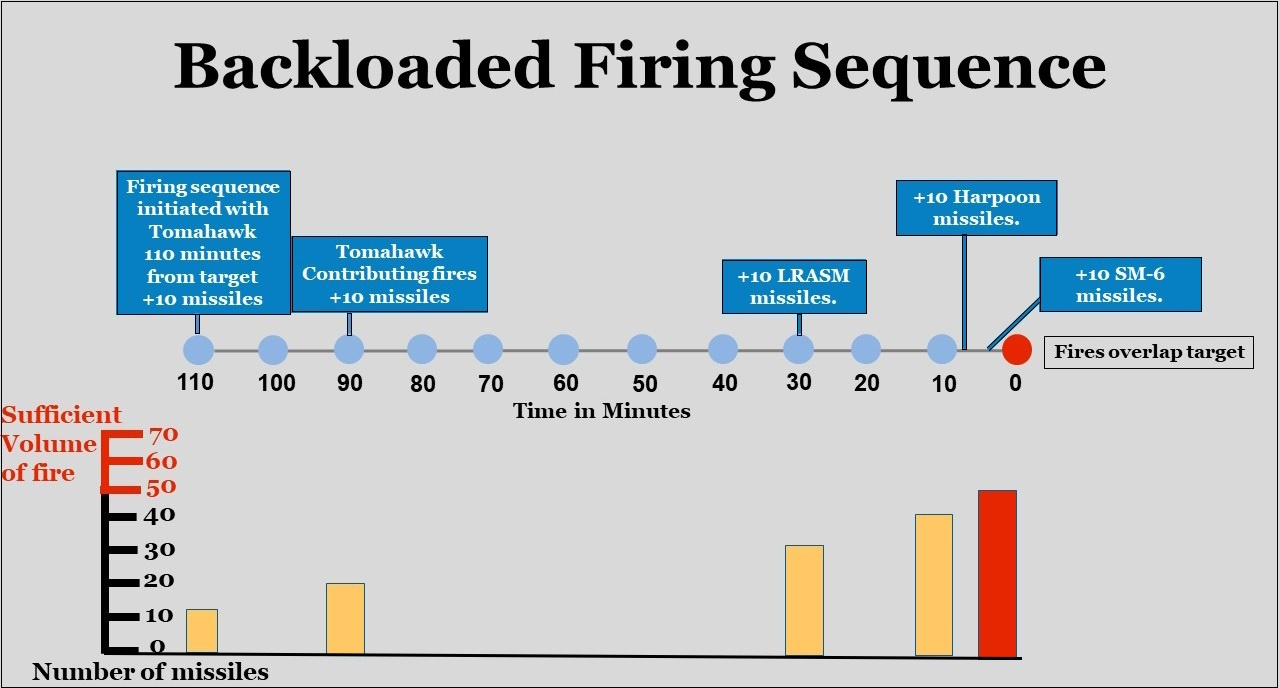

These dynamics create a conundrum for using higher-end weapons. These weapons typically feature very low flight times by virtue of their especially high speed. Their speed will often place them later in the firing sequence where they can combine with more common weapons over the target. Using higher-end weapons is therefore more likely to backload the firing sequence of a mixed salvo. Since the weapons that could contribute the most to a salvo’s lethality would often be fired last, this creates more dependence on ensuring those forces and their kill chains survive until the final minutes of a firing sequence. If those platforms are destroyed or suppressed, or if the handful of high-end missiles are shot down by defenses, then the rest of the aggregated salvo may be at risk of failing and with virtually no time left to add more contributing fires. Counting on higher-end missiles to push a mixed salvo into overwhelming dimensions near the very end of a firing sequence leaves little room to recover lost volume during the course of the attack.
Commanders may not want to risk these dependencies. Therefore they may opt to shorten the overall length of the firing sequence, such as by firing salvos that mainly consist of higher-end weapons. Firing salvos primarily of the fastest weapons will shorten the decision cycle considerably compared to having to wait tens of minutes or longer for more common weapons to form massed fires. A greater number of firing sequences and mass firings could take place within the same span of time it takes to launch a single slower salvo. More than 20 consecutive SM-6 strikes or seven DF-21 anti-ship ballistic missile strikes could be conducted within the time it takes a single Tomahawk salvo to travel the limits of its range (Figure 15). This assumes of course that enough SM-6 and DF-21 inventory is available, targeted, and ready to fire.
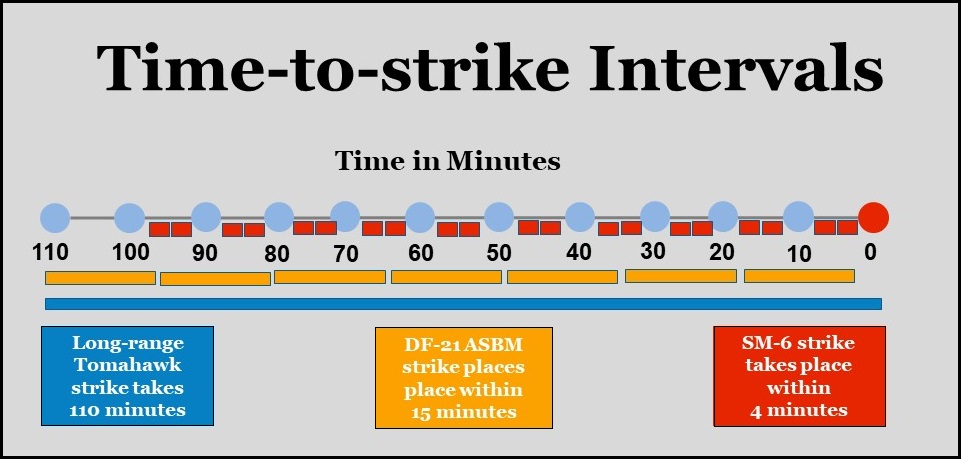
Faster weapons can result in a faster kill chain and increase decision-making advantage. A faster kill chain creates more opportunity to launch more attacks, adjust volumes of fire as needed, improve understanding of adversary defenses, and move on to new targets. These advantages may come at a steeper logistical price by depleting high-end inventory at a faster rate. Yet distributed forces that heavily depend on more common weapons with long flight times, like the Tomahawk, may suffer considerable disadvantage in the speed of their decision cycle.
Massing Fires with Aviation
These frameworks for assembling massed fires presume a relatively static laydown of forces from the start to finish of a firing sequence. This is a fairly reasonable assumption when missiles can travel hundreds and even thousands of miles within timeframes that a ship or land vehicle can travel only tens of miles. Most launch platforms will have to rely on the speed and range of their missiles to compensate for their platform’s lack of near-term maneuver in a missile exchange.
Aviation is a critical exception to this. Aviation is the only launch asset whose speed can approach and even exceed that of cruise missiles. The scope of a weapon’s reach can be greatly enhanced by the speed and range of aerial launch platforms, where aviation can put fires in many more places than warships can with similar-ranged weapons in similar timeframes. Through speed and maneuver, aviation can be dynamically repositioned to bolster aggregated salvos in tactically meaningful timeframes. This ability to add flexible on-demand fires makes aviation an especially potent force multiplier for distribution and aggregation. But leveraging aviation poses challenges for assembling massed fires.
First, an important contrast has to be drawn between the availability of fires from carrier air wings, warships, and bombers. One critical advantage carrier aviation has over warships in launching anti-ship strikes is logistics. Carriers have especially deep magazines, and air wings can be rearmed in a matter of hours compared to the days or weeks it can take to rearm warships exiting the theater. But it is quite possible that air wings cannot be armed and sortied quickly enough to satisfy pressing operational demands in a shorter timeframe, such as fitting into a tight firing sequence. It can take a considerable amount of time to finalize mission planning for a large airborne strike, arm dozens of aircraft with specific weapon loadouts, launch those aircraft, assemble the air wing in flight, and then prosecute the strike.7 Aviation-based fires cannot be contributed until planes are loaded and made airborne.
While warships cannot rearm cruise missiles at sea like an air wing can, aviation cannot always match the promptness of warship-launched fires. By fielding weapons within launch cells, warships can fire salvos relatively soon after the decision is made to strike, essentially bypassing some of the steps it would take to deliver similar firepower through aviation.8 Commanders attempting to combine fires from carrier aviation and warships may find the near-term time demands of setting up aviation are constraining quicker options for massing fires. Commanders in need of rapidly deployed firepower may very well opt for warship-based fires over aviation-based ones, and be willing to pay the steeper logistical price of depleting warships in exchange for the earlier application of firepower.
It may be too logistically taxing to keep most of a carrier air wing airborne and on station for the sake of maintaining quicker options for fires. Instead, it is more likely that a carrier air wing would be armed and launched once targets have been definitively selected and the strikes ordered. If enough anti-ship firepower is widely fielded to the point that entire air wings are not necessary to achieve volume of fire, then smaller numbers of carrier aircraft can contribute a fraction of the contributing fires and reduce the time required to prepare aerial strikes. But compared to carrier aircraft, bombers offer a much more stable and enduring source of on-station aerial firepower by virtue of their longer endurance. This on-station endurance can allow bombers to provide options for fires that are more quickly deployed than air wings that need time to prepare and get airborne for massed strikes. The following schemes of assembling massed fires with aviation are more feasible with heavy bombers than full carrier air wings.
Combining fires between ships and aircraft will often depend on how much repositioning aviation needs to set up its contributing fires. But repositioning costs time, where taking advantage of aviation’s high speed to bolster salvos on demand will cost the time it takes to use that speed. That time is also needed to use speed to compensate for how U.S. aircraft are often limited to carrying smaller and shorter-ranged cruise missiles than the ship-launched weapons they can be combining fires with.
The time it costs to reposition aviation can delay massed fires, put aviation later in the firing sequence, and force other platforms to wait on aircraft to move. Flexible repositioning is one of aviation’s greatest potential contributions to massed fires, yet the time it costs to reposition can complicate aggregation and firing sequences. A critical question is how to position aviation in advance to create options for quick and flexible fires.
The extent to which warships are forced to wait on aviation depends on aviation’s position relative to the target and to the friendly warships they are combining fires with. The extent to which aviation will need to reposition after warships initiate the firing sequence mainly depends on aviation’s proximity to the target. Simply put, how do things change if aviation is kept on station in the space between opposing fleets, or when aviation is kept behind friendly fleets?
If aviation is kept behind friendly warships, then warships will often have to wait until enough aviation is assembled and then maneuvered across lines of departure before the warships can initiate the firing sequence with their longer-ranged weapons. Those aircraft may then have much of their ability to maneuver on the way to the target tightly constrained by the need to adhere to the timing of the firing sequence while still having to travel hundreds of miles forward to their launch points.
If aviation is maintained in the space between opposing fleets, then warships can initiate massed fires without having to wait as much for aviation to reposition. In this scheme, the need to reposition aviation can be deferred to the point of it not being a hard prerequisite for initiating the firing sequence. Aviation would have more flexibility to maneuver as needed while the firing sequence is in progress, rather than be locked into a more constrained flight path from the outset and across a longer distance.
Maintaining aviation in the space between opposing fleets will allow massed fires to be initiated earlier. But aviation positioned in this space may be deprived of the valuable air defense and sensing support that friendly warships can provide. It can also be more risky to maintain an aloft presence with aerial tanking in such a forward position, and protecting strike aircraft in a forward position could create substantial air defense requirements for carrier air wings and other aircraft. But unless aviation has missiles with similar ranges and flight times as the larger warship-based weapons, a force that wants quicker options for massing firepower will accept more risk to aviation by maintaining aerial presence in the space between opposing fleets.
Regardless of where they are maintained in the battlespace, once strikes are ordered, aviation will often need to go far beyond the protections of friendly warships that can fire from much longer standoff ranges. If a bomber with LRASM needs to combine fires with a nearby warship’s 800-mile-long Tomahawk strike, that bomber could have to travel 500 or more miles deeper into the contested battlespace before it can launch its own weapons. While other contributing salvos are in flight, aviation will have to be traveling deeper into the battlespace until the necessary time factors overlap so they can add their own fires. This challenge can be greatly mitigated by fielding larger or more capable cruise missiles that can shift more burden of maneuver from the platform to the payload, such as by equipping bombers with Tomahawks or extreme-range JASSMs. This would allow aviation to fire from more flexible standoffs ranges that are comparable to that of warships.
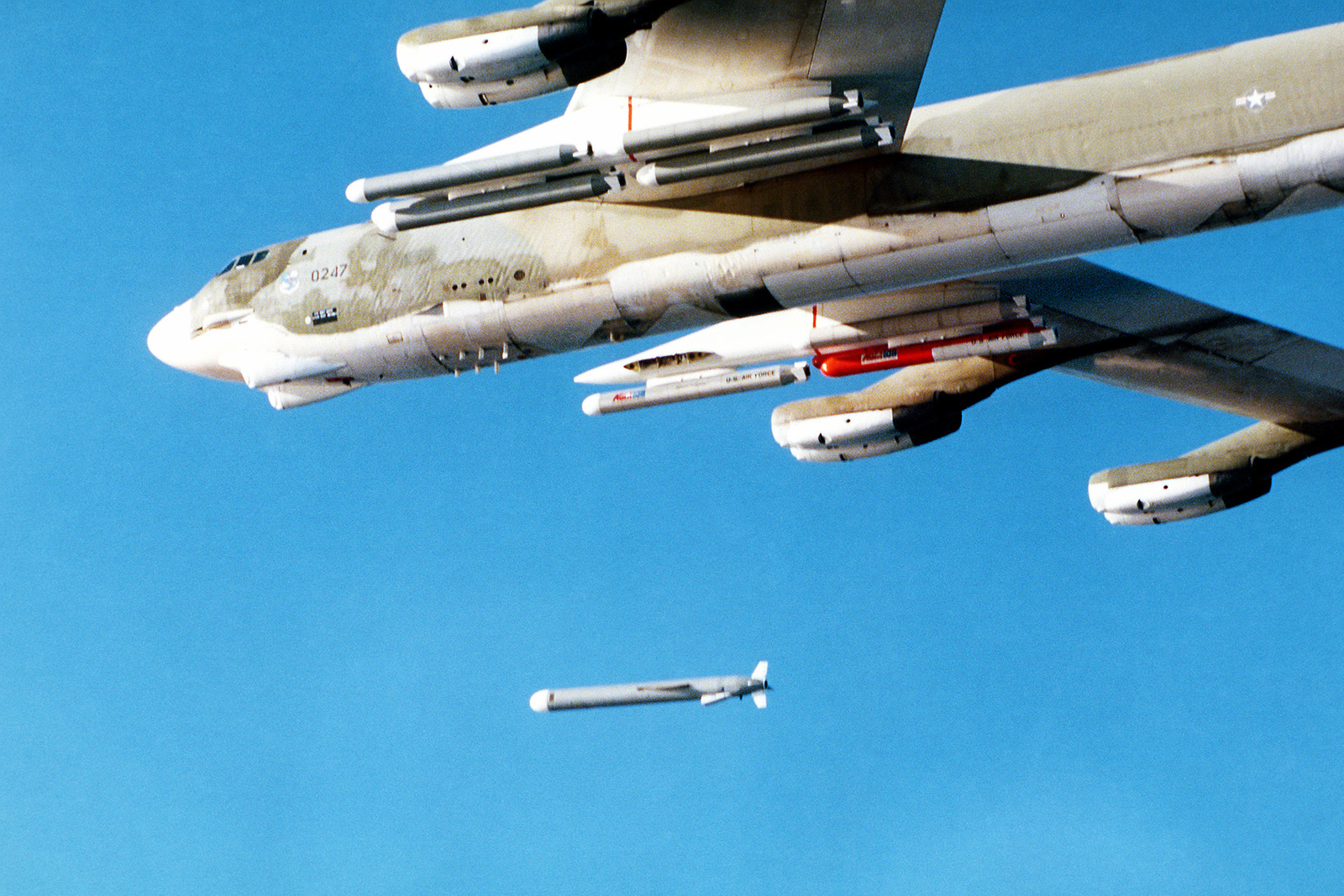
The disposition of aviation would be constrained by the relationship between the speed of the aircraft and the speed of the missiles they are combining fires with. In the U.S. military, many of the bombers and cruise missiles have similar subsonic speeds. Subsonic bombers like the B-52, B-2, and B-21 have fewer options for aggregating with subsonic salvos than faster aircraft. Aircraft that can outpace subsonic missiles, such as strike fighters and B-1 bombers, could be held further back and across wider distributions. If commanders are willing to pay the logistical price, they can use supersonic flight to surge these aircraft forward in time to combine fires with slower subsonic salvos.
Conclusion
Assembling massed fires from distributed forces will be a complicated challenge. It will involve mixing and harmonizing the kill chains of different payloads, platforms, communities, and services. Each of these factors comes with a variety of its own dependencies and pitfalls. As the services look to operationalize mass fires, they must be mindful of how too much complexity and too much sensitivity to tight coordination can threaten to yield brittle operational designs.
Part 4 will focus on weapons depletion and the last-ditch salvo dynamic.
Dmitry Filipoff is CIMSEC’s Director of Online Content and Community Manager of its naval professional society, the Flotilla. He is the author of the “How the Fleet Forgot to Fight” series and coauthor of “Learning to Win: Using Operational Innovation to Regain the Advantage at Sea against China.” Contact him at Content@Cimsec.org.
References
1. The full quote is as follows: “At sea better scouting – more than maneuver, as much as weapon range, and oftentimes as much as anything else – has determined who would attack not merely effectively, but who would attack decisively first.”
See:
Wayne P. Hughes, Jr., Fleet Tactics: Theory and Practice, Naval Institute Press, pg. 173, 1986.
2. For an example on the need of very close timing for a mass firing sequence of anti-ship missiles, see:
Maksim Y. Tokarov, “Kamikazes: The Soviet Legacy,” U.S. Naval War College Review, Volume 1, 67, 2014, pg. 17, https://digital-commons.usnwc.edu/cgi/viewcontent.cgi?article=1247&context=nwc-review.
3. This flight time is derived from an estimate of a maximum missile speed of 550mph, or about 9.16 miles per minute, and applying this speed to a maximum missile range of 350 miles.
4. For weapon range, see:
“Options for Fielding Ground-Launched Long-Range Missiles,” Congressional Budget Office, pg. 24, 2020, https://www.cbo.gov/publication/56143.
For 550mph subsonic speed of Tomahawk, see:
“Beyond the Speed of Sound,” pg. 158 (PDF page 166), Arnold Engineering Development Center’s contributions to America’s Air and Space Superiority, United States Air Force, https://www.arnold.af.mil/Portals/49/documents/AFD-100322-069.pdf.
5. This estimate is based on the typical flight times of similar intermediate range ballistic missiles. See:
Bruce G. Blair, Harold A. Feiveson and Frank N. von Hippel, “Taking Nuclear Weapons off Hair-Trigger Alert,” Scientific American, November 1997, https://sgs.princeton.edu/sites/default/files/2019-10/blair-feiveson-vonhippel-1997.pdf.
Dr. Jamie Shea, “1979: The Soviet Union deploys its SS20 missiles and NATO responds,” NATO, March 4, 2009, https://www.nato.int/cps/en/natohq/opinions_139274.htm.
Charles Maynes, “Demise of US-Russian Nuclear Treaty Triggers Warnings,” Voice of America, July 31, 2019, https://www.voanews.com/a/usa_demise-us-russian-nuclear-treaty-triggers-warnings/6172981.html.
6. This estimate is derived from the flight times listed in Figure 1, where SM-6 has four minutes of flight time, and a Tomahawk missile has a maximum flight time of 110 minutes.
7. For comparisons of times to plan and launch Tomahawk versus carrier air wing strikes, see:
General Accounting Office, “Cruise Missiles: Proven Capability Should Affect Aircraft and Force Structure Requirements,” GAO/NSIAD-95-116, April 1995, pg. 35-36, https://www.gao.gov/assets/nsiad-95-116.pdf.
8. General Accounting Office, “Cruise Missiles: Proven Capability Should Affect Aircraft and Force Structure Requirements,” GAO/NSIAD-95-116, April 1995, pg. 35-36, https://www.gao.gov/assets/nsiad-95-116.pdf.
Newer Tomahawk variants than those discussed above have considerably shorter launch preparation times. See:
“Tomahawk,” Missile Threat CSIS Missile Defense Project, last updated February 23, 2023, https://missilethreat.csis.org/missile/tomahawk/
and
Rear Admiral Edward Masso (ret.), “On The Tomahawk Missile, Congress Must Save The Day,” Forbes, June 10, 2015, https://www.forbes.com/sites/realspin/2015/06/10/on-the-tomahawk-missile-congress-must-save-the-day/?sh=7b86cc956bad.
Featured Image: PACIFIC OCEAN (August 17, 2018) The guided missile destroyer USS Dewey (DDG 105) conducts a tomahawk missile flight test while underway in the western Pacific. (U.S. Navy photo by Mass Communication Specialist 2nd Class Devin M. Langer)