By Lieutenant Kyle Cregge, USN
The US Navy’s strike capacity is shrinking. As highlighted in Congressional testimony with senior leaders, the Surface Navy is set to lose 788 Vertical Launch System (VLS) cells through the end of the Davidson Window in 2027. This 8.85% of current Surface Navy VLS capacity represents the equivalent of eight Arleigh Burke-class destroyers leaving the fleet as the Ticonderoga cruisers are retired. However, even the most aggressive and expensive shipbuilding alternative would not return equivalent VLS numbers to the surface fleet until the late 2030s. Present maritime infrastructure capacity further strangles efforts to buy additional Arleigh Burke destroyers, Constellation-class frigates, and Virginia-class submarines. These complex multi-mission ships cost billions of dollars and years of investment in build times, and yet service life extension proposals are equally unsavory. From extending aging Ticonderoga cruisers to arming merchants or Expeditionary Fast Transports, none are cheap, scalable, or sustainable in the long-term. All this while the world’s largest navy, the People’s Liberation Army Navy (PLAN), continues its building spree at speed and scale, delivering combatants equipped with long-range anti-ship missiles meant to challenge America’s role as balancer in Eurasia.

Where can the Surface Navy focus its efforts for future growth given the financial constraints and maritime industrial base capacity? What capabilities are most likely to enable a replaceable, lethal force to deter or deny Chinese aggression from the Taiwan Strait to the Second Island Chain?
The Surface Navy must build and deploy the Large Unmanned Surface Vehicle (LUSV) at scale as small surface combatants, to economically restore and grow VLS capacity over the next decade. A concept for its implementation and other USVs like it, “Every Ship a SAG,” proposes a distributed future force architecture, where every manned ship can operate far afield from each other, while each is surrounded by multiple VLS-equipped and optionally manned LUSVs. Doctrinally, a Surface Action Group (SAG) is defined as a temporary or standing organization of combatant ships, other than aircraft carriers, tailored for a specific tactical mission. Together, these manned-unmanned teams will form more lethal SAGs than a single ship or manned surface action group operating alone. Led by Surface Warfare Lieutenants as Unmanned Task Group Commanders, this USV-augmented SAG offers a lethal instantiation of the next-generation hybrid fleet.
“Every Ship a SAG” provides a scalable and flexible model for incorporating current and future unmanned systems with the existing surface fleet. The fleet could rapidly up-gun conventional platforms and even amphibious ships, Littoral Combat Ships (LCS), or Expeditionary Staging Bases (ESB) with more lethal USVs as teammates. Lastly, “Every Ship a SAG” offers mitigation for many of the concerns levied at Navy USV concepts, including Hull, Mechanical, and Electrical (HM&E) reliability, maintenance, and spare parts; force protection; C5I/Networks; autonomy; and the role of USVs in deterrence. Mutual support from a manned ship reduces operational risk and will enable the small crew led by the Surface Warfare Early Commander to embark on their USV to execute critical manned operations during dangerous or restricted waters evolutions. These small teams then debark to a designated mothership and perform USV mission integration when the USV is in an unmanned mode. “Every Ship a SAG” offers a critical next step between today’s nascent USV capability and a more advanced, USV-forward, and independent future.
Now is a critical moment in history. LUSVs must be scaled to meet the Navy’s warfighting mission, and Congress must resource the supporting pillars to ensure effective outcomes. When every manned US Navy ship is a Surface Action Group, this distributed hybrid fleet will be more lethal, survivable, and ready to fight and win maritime wars against peer adversaries.
Defining “Every Ship a SAG”
The Secretary of the Navy and the Chief of Naval Operations have consistently argued for the introduction of unmanned systems and their incorporation into the fleet. Leaders have envisioned LUSV as a 200-300ft low-cost, high endurance, and reconfigurable corvette accommodating up to 32 VLS cells. The ship is programmed to be bought in Fiscal Year 2025 with subsequent buys out to 2027 with a three-ship purchase at $241 million per ship. The Navy’s unmanned strategies have referred to LUSVs as “adjunct magazines,” providing greater strike and anti-surface warfare weapons. This vision is appropriate, but has narrowly scoped the ship’s offensive technical capabilities. Myriad experts have penned compelling, lengthy vignettes illustrating USVs in the fleet, with advantages including sensor networking, depth of fire, survivability, and many others.
The “Every Ship a SAG” construct offers a vision for weaponized USVs that is easily understood; from the average fleet sailor to senior leaders to (maybe most critically) Congress. In addition, the concept acknowledges the current fleet design both in Strike Groups and Surface Action Groups, while facilitating the introduction of unmanned ships within a task organization framework common to manned units. Operationally, LUSVs will meet specific, near-term needs in support of national strategies via distributed sea denial and strike, while enhancing the lethality of the surface fleet through increased missile magazine distribution and capacity. When integrated into the force, LUSVs will increase the survivability of the fleet by complicating an adversary’s ability to target and attack surface forces. What does this look like in practice?
In a peacetime environment and workup cycle, the Unmanned Operations Center (UOC) and USV Divisions in Port Hueneme, California, or a local Fleet Maritime Operations center, would manage the traditional “manning,” training, and equipping functions of ship workup cycles towards integrating into Strike Groups and SAGs. These LUSV Divisions would be led by Early Command Junior Officers. In fact, the Surface Community has already begun selecting officers for Unmanned Task Group Early Command roles both in Port Hueneme and in Bahrain with Task Force 59.
Having been assigned to units for scheduled deployments, LUSVs would attach to the designated ships in the deployment group, providing greater flexibility to Combatant Commanders in force packages. Just as the MH-60 Romeo community deploys expeditionary detachments of pilots and aircrew to cruisers and destroyers, these Early Command officers and a small crew would embark a ship, or series of ships, serving in a variety of modalities as expert controllers, emergency maintainers, and expeditionary operators. A key distinction between the helicopter detachment concept and command is the interchangeability of USVs, moving from independent expeditionary command with a manned crew, to embarking on a mothership or series of motherships supporting unmanned operations.
Figure 2: A top-level view comparing USV employment models with generalized benefits and limitations. (Author-generated graphic)
As demonstrated in Figure 2, LUSVs would operate at distances where the manned ship can provide mutual support and respond if needed. This might include periods within the visible horizon but also episodic surges well over the horizon for specific missions. From a lethality perspective, the additional VLS cells and sensors (in the Medium Unmanned Surface Vehicle) offer enhanced battlespace awareness and depth of fire than is available with a single ship. While others have argued for pushing attritable USVs far forward towards threats, treating every manned ship as a SAG with its LUSVs in escort will address many of the issues highlighted by leaders, including Congressional representatives.
Concerning reliability and maintenance, the Navy has based LUSV prototypes on existing commercial ship designs while conducting further land and sea-based testing and validating its critical technologies and subsystems. While designed to operate for extended periods without intervention, the Unmanned Expeditionary Detachment will be able to support emergent repair or troubleshooting if necessary.
For concerns of autonomy or ethical use of weapons from unmanned units, LUSVs will rely on human-in-the-loop (HITL) for command and control of weapons employment decisions. Therefore an on-scene commander simplifies network and communications requirements between the manned fleet and its LUSV escorts. Others have also argued for unmanned systems to be attritable, and to be sure, it would be preferable to lose an LUSV to a manned ship. However, these will still be multi-million dollar combatants with exquisite technology that should not fall into an adversary’s hands – much in the same way how Fifth Fleet dealt with Iranian attempts to capture a US Saildrone in 2022. Having a local manned combatant nearby will support kinetic and non-kinetic force protection of the LUSV, regardless of the theater or threat.
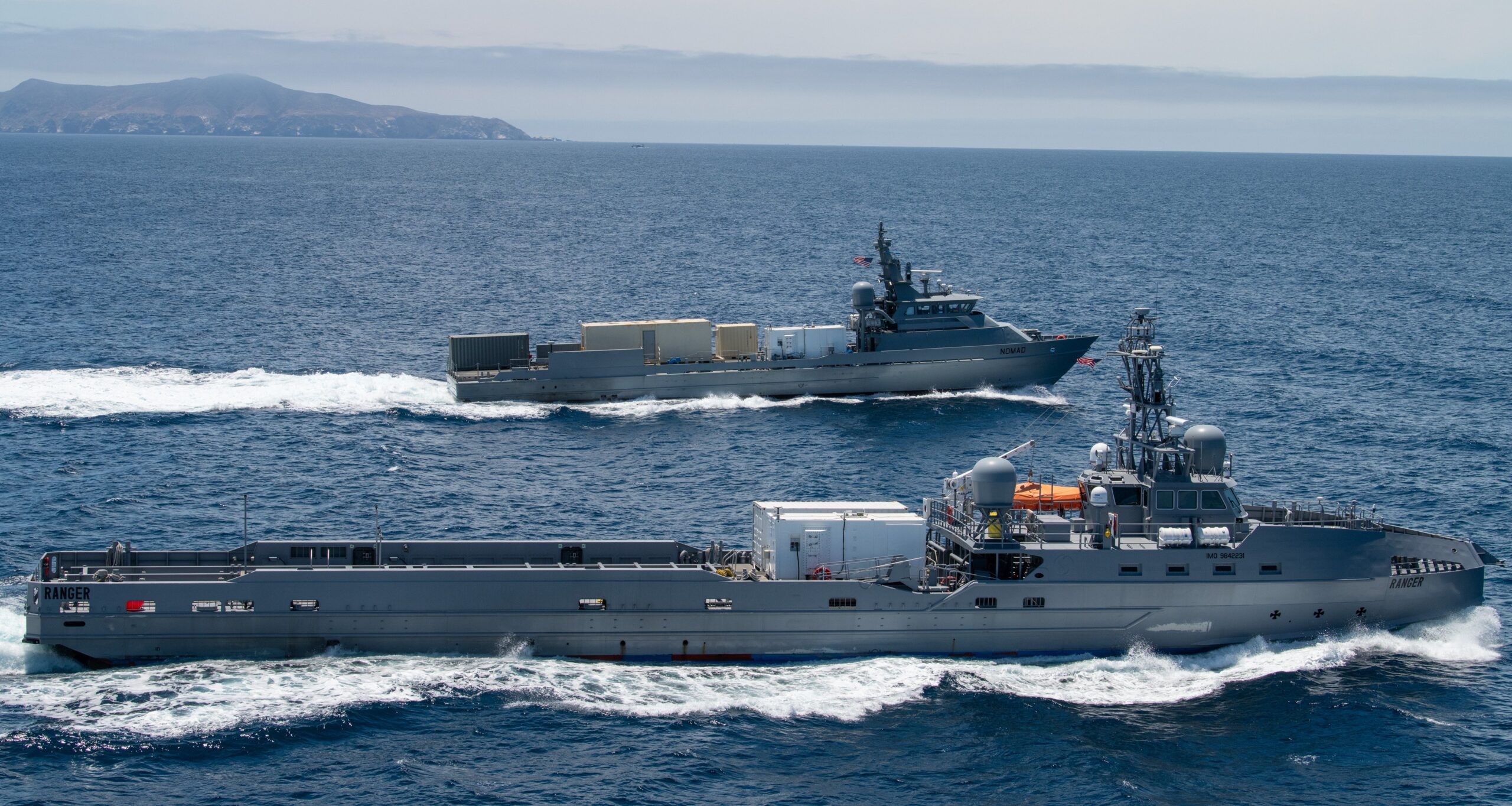
Finally, treating an LUSV as a force multiplier with a certain number of VLS cells is in line with previous arguments to count the fleet via means other than ship hulls, and simplifies the LUSV’s deterrent value as just another ship that delivers a specific capability at a discount, just as other manned ships do.
Sequencing and Scaling “Every Ship a SAG”
No vision for USV integration into the Surface Force would be complete without considering how these systems would fit into the career pipeline of current and future Surface Warfare Officers and their enlisted teams. In an “Every Ship a SAG” model, LUSV ships would start as individual early commands for post-Division Officer Lieutenants, whereas multiple LUSVs would be organized into a Squadron, led by a post-Department Head Early Command Officer. The Surface Community executed this model with its Mark VI Patrol Craft before their recent retirement, and similarly these squadrons would be organized under the nascent USV Divisions, who have a direct line to the experimentation and tactical development done by the Surface and Mine Warfighting Development Center (SMWDC), and specifically for unmanned systems, in Surface Development Squadron One (SURFDEVRON).
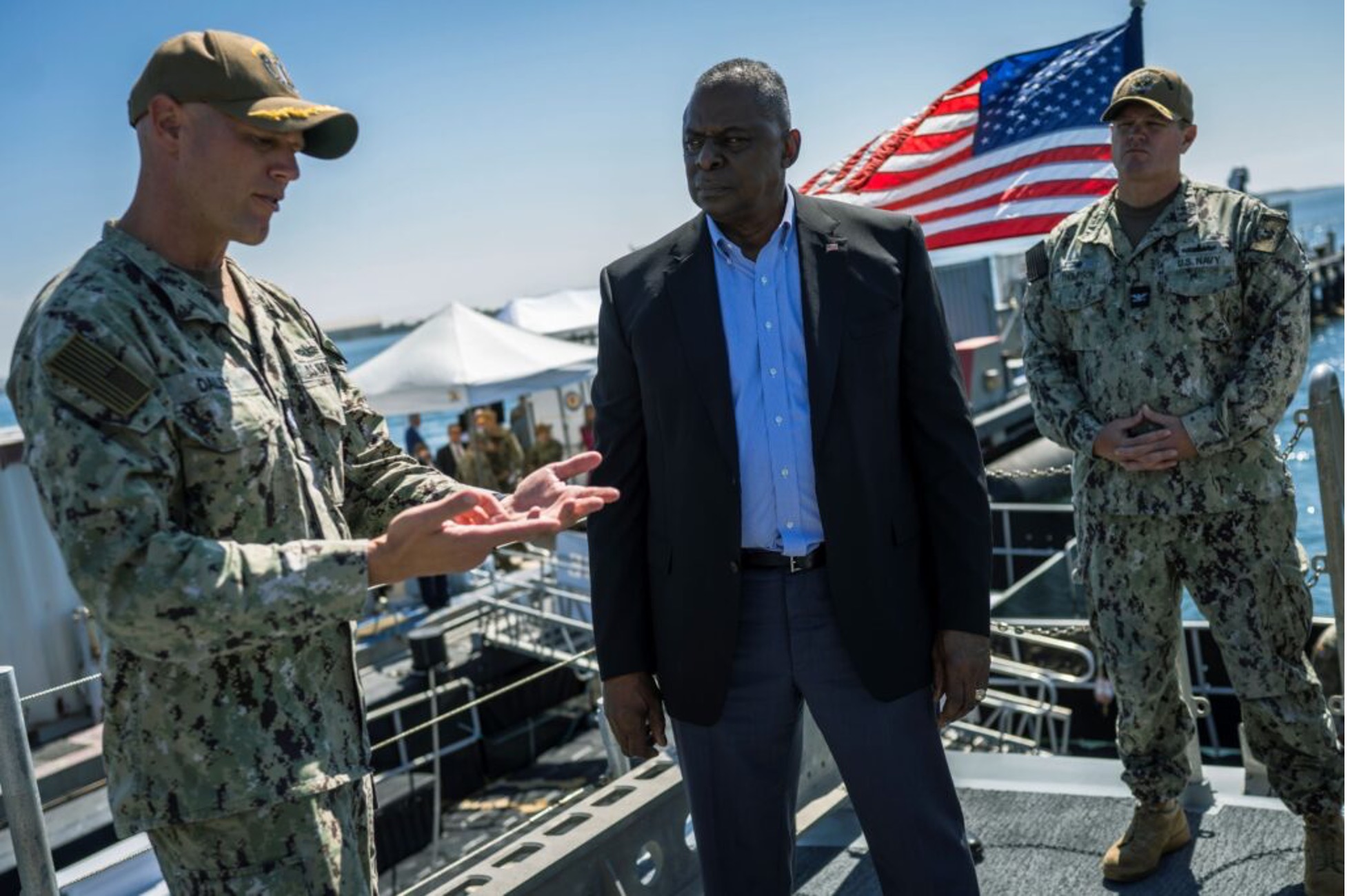
The surface community is leading the charge towards a hybrid fleet by advancing USV operational concepts and integrating unmanned experience into a hybrid career path. The first salvo in this career movement was launched in 2021, with the establishment of the Unmanned Early Command positions, but scaling this hybrid model is both critical and beneficial. The community will only benefit from commanding officers with expertise and insights in employing a hybrid surface fleet. As pipelines are clarified and unmanned opportunities grow, officers would transition from one expeditionary tour leading a detachment controlling and maintaining an LUSV, back into Division Officer, Department Head, Executive, and Commanding Officer roles in traditional at-sea commands directing the employment of the same LUSVs. Just as the SWO Nuke community develops expertise in both conventional and nuclear fields at each level of at-sea tours, a future hybrid fleet necessitates competencies in fields like robotics, engineering, applied mathematics, physics, computer science, and cyber.
Lastly, SWO professional experiences and investments in training and education for the use of unmanned systems would further Navy and Department of Defense objectives around Artificial Intelligence, Big Data, and Digital Transformation. With unmanned systems, deploying new HM&E or weapons payloads may be a simpler task compared to accelerating fleet data collection and its subsequent use in software development and delivery. Task Force 59 explicitly linked these issues as the Fifth Fleet Unmanned and Artificial Intelligence Task Force.
“Every Ship a SAG” on a Digital Ocean
Some may question whether “Every Ship a SAG” aligns with the already successful work of Task Force 59, directed by Vice Admiral Brad Cooper, Commander, Naval Forces Central Command, and Captain Michael Brasseur, the Task Force’s Commodore. Captain Brasseur has long advocated for increased AI and Unmanned Integration into the Navy, going back to his time as Co-Founder and first Director of NATO’s Maritime Unmanned Systems Innovation and Coordination Cell (MUSIC^2). He convincingly argued for a “Digital Ocean” Concept where drones:
“Propelled by wind, wave, and solar energy… carry sensors that can collect data critical to unlocking the untapped potential of the ocean…. [to] exploit enormous swaths of data with artificial intelligence- enhanced tools to predict weather patterns, get early warning of appearing changes and risks, ensure the free flow of trade, and keep a close eye on migration patterns and a potential adversary’s ships and submarines.”
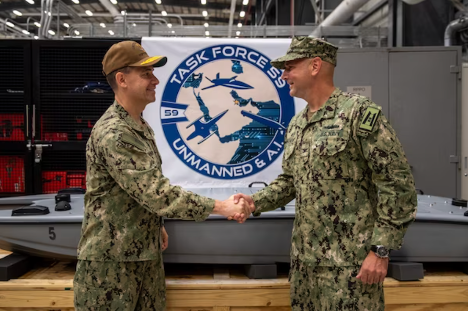
Captain Brasseur has implemented his prudent and innovative vision in the Fifth Fleet Area of Responsibility. Task Force 59 is a success whose model is likely to be adopted in other theaters. Rather than conflict with the “Digital Ocean” model, “Every Ship a SAG” complements this work in line with missions of the US Navy as Congressman Mike Gallagher recently updated and codified in the 2023 National Defense Authorization Act. The Wisconsin Representative edited the Title 10 mission of the Navy such that the service “shall be organized, trained, and equipped for the peacetime promotion of the national security interests and prosperity of the United States and prompt and sustained combat incident to operations at sea.” In short: a “Digital Ocean” and all it enables serves the peacetime promotion of American national security interests and prosperity, especially in coordination with our allies and partners.
“Every Ship a SAG” postures the Navy for prompt and sustained combat operations incident to the sea. Both missions have been a part of the U.S. Navy since its inception, and both visions are applicable as unmanned ships enter our fleets. Further, LUSVs retain additional utility below the level of armed conflict. To support UOC training, experimentation, and manned ship certifications, LUSVs would serve as simulated opposition forces during high-end exercises, reducing demand on manned sustainment forces, or enabling higher-end threat presentations. Precisely in these scenarios are the venues whereby the fleet can integrate new systems and networks while bridging toward operational concepts for unmanned systems as LUSVs earn increased confidence. In the interim and foreseeable future, however, “Every Ship a SAG” remains the scalable, flexible model for deployed LUSVs within current fleet operations.
Sober Acknowledgement of Critical Pillars
Unmanned ships and various other transformational technologies are not a panacea for the current and future threats facing the US Navy. Even the promises and methodologies proposed here rely upon critical readiness pillars, each of which could warrant deep individual examinations but are worth mentioning.
Even if the US Navy built a certain number of LUSVs to replace lost VLS capacity, failure to resource them or manage them effectively would still likely doom the program. The fleet must understand and plan for the “total cost of ownership” of a hybrid fleet. These units will still require manpower at various levels and a maintenance infrastructure to sustain them in fleet concentration areas. Nor can the fleet avoid at-sea time to test, integrate, and experiment with these systems, much in the same way that RADM Wayne E. Meyer emphasized, “build a little, test a little, learn a lot,” with the success of the Aegis Weapons System. The Navy has made efforts to assuage Congressional concerns about reliability through investment in land-based testing. Yet the Surface Navy will need continued, reliable resourcing to continue that testing afloat while integrating LUSVs with traditional forces and experimenting with future concepts.
Characterizing those costs are beyond what is available in open-source, but wide-ranging demand for talent is imposing costs across the public and private sectors. Similarly dire is the state of munitions, as highlighted at the Surface Navy Association National Symposium by Commander, Fleet Forces Command, Admiral Caudle who “noted that [even] if the Navy had ready its 75 mission-capable ships, ‘their magazines wouldn’t all be full.’” Put simply: no amount of LUSVs built at economic costs will be worth anything if they lack the appropriate weapons to place in their launchers.
Lastly, the adaption of agile practices to implement better software, data, AI models, etc., is critical for the fleet to field increasingly capable and autonomous USVs. The Department of Defense and the Navy have made various investments in this direction. These include but are not limited to the Program Executive Office for Integrated Warfare Systems (PEO IWS) “The Forge” working to accelerate ship combat system modernizations and development of the Integrated Combat System; to the Naval Postgraduate School’s new Office of Research and Innovation, to the type-command AI Task Forces. Each is working to provide value across various programs in the digital space. Resourcing, integration, and acceleration of those efforts are crucial.
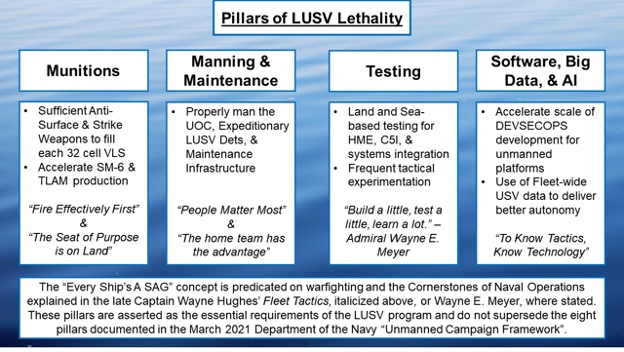
Individually, each pillar is a wicked problem, but we must take a sober look at those requirements while examining the same realities in the maritime industrial base. The reality appears that little can be done in the near term to accelerate new ship deliveries of complex multi-mission combatants built in Bath, Maine, and Pascagoula, Mississippi. At present, Fincantieri Marine in Wisconsin is the sole yard for FFG-62, while the remaining large shipyards pursue some collection of ESBs, littoral connectors, and generally, more multi-mission units. Fundamentally, a ship like LUSV is the only near-team option to accelerate a pre-war ship buildup given the PLAN’s construction speed.
As the world’s only Navy with a near-term plan and resourcing to meet and exceed 355 ships, the PLAN along with its fellow services has delivered longer-range weapons at greater capacities than the United States for years. By all available open-source data, the US Navy is falling behind the PLAN in the marathon of naval power while the PLAN accelerates toward future advantages.

Naval writers and thinkers can parse arguments about quantity versus quality, what the right metric is to assess fleet strength, or whether in a joint, Navy vs. Anti-Navy fight, a pure-maritime comparison is warranted. These are valuable discussions. Regardless, the US Navy’s Surface Forces onboard strike and anti-surface warfare capacities will continue to shrink in the near-term while Chinese threats accelerate. Furthermore, the Chinese industrial base capacity far exceeds American capacity at present. The relationship between US Navy leaders and industry could be described as frosty at best, with recent comments from the Chief of Naval Operations to industry including statements to “Pick up the pace… and prove [you have extra capacity]” and from the Commander of Fleet Forces Command stating that he is “not forgiving” industry’s delays.
Given the long-term buys of multi-mission combatants, national shipyards appear unlikely to generate increased efficiencies, accelerated timelines, or better-quality ships if they continue to build only the multi-billion dollar multi-mission combatants they have previously built. Accelerating LUSV procurement across the six shipyards solicited for LUSV concepts would provide increased capital and demand signal for the shipbuilding industry while providing complementary capabilities to the fleet. Yet while the LUSV can and should be a domestic program for growth, corvette-sized unmanned ships with VLS could easily fall into cooperative build plans with the various allies and partners who have frigate-sized, VLS-equipped combatants. The Australia-United Kingdom-United States (AUKUS) technology-sharing agreement could provide an additional avenue for foreign construction. Further US coordination with Japan and South Korea could also prove fruitful, as the two East Asian allies represent the second and third largest global commercial shipbuilders behind China.
While refining broader LUSV programs, it is worth considering the differences in shipbuilding costs between choosing LUSVs in a SAG compared to traditional manned combatants. Figure 5 provides a table of notional Surface Action Groups based on the fleet of today through 2027, while Figure 6 presents a table with the future ship programs and their costs.
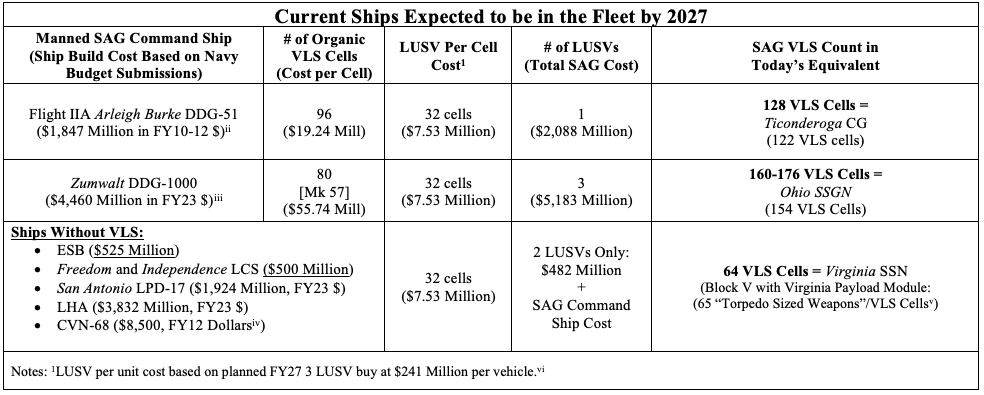

Congressional Budget Office estimates for future programs like SSN(X) and DDG(X) present stark realities. The next-generation programs could run costs up to $6.3 billion and $3.3 billion, respectively. By comparison, if the Surface Navy chose to pursue an expanded LUSV buy to recapitalize the 788 VLS cells planned to disappear through 2027, this would require 25 32-cell LUSVs, totaling 800 cells. At $241 million per LUSV, the total (shipbuilding-only) costs would be $6.025 billion, or approximately less than a single SSN(X) or two DDG(X)s. While LUSV has a reduced collection of mission sets by comparison to future submarines and destroyers, it remains a ship that can conceivably be built in at least six American shipyards. Further, future LUSVs purpose-built to support Conventional Prompt Strike (CPS) could hypothetically resolve the issue of the margin of the DDG-51 hull form being “maxed out” in space, weight, air, power, and cooling. Rather than a future large surface combatant required to have each capability resident in a single hull, as in DDG(X), a CPS LUSV in escort with a Flight III DDG may represent a proven ship design and better value, that other companies are attempting to support.
Ultimately, there are myriad ways to frame budgetary realities, but LUSV is the only cost-effective method for the surface force to quickly scale VLS capacity within existing force structure and given the present maritime industrial base.
Conclusion
The Surface Navy has a crucial opportunity to strengthen its capabilities and enhance its readiness by building and deploying LUSVs at scale. The “Every Ship a SAG” concept remains rooted in the intellectual work going back nearly a decade to “Distributed Lethality,” “Hunter-killer SAGs,” and their incorporation into Distributed Maritime Operations – only now with unmanned combatants. This manned-unmanned model provides a feasible solution for incorporating unmanned systems into the Surface Warfare Officer career path and forming more lethal Surface Action Groups for the future fight.
“Every Ship a SAG” addresses the concerns raised about Navy USV concepts and presents a clear vision for the future of wartime maritime operations. As the global security situation continues to evolve, the Surface Navy must take decisive action and invest in LUSVs to ensure it is prepared to meet its warfighting mission. It is time for Congress to fully support this effort by providing the necessary resources to bring the “Every Ship a SAG” model to life. Act now and make every ship a Surface Action Group.
Lieutenant Kyle Cregge is a U.S. Navy Surface Warfare Officer. He is the Prospective Operations Officer for USS PINCKNEY (DDG 91). The views and opinions expressed are those of the author and do not necessarily state or reflect those of the United States Government or the Department of Defense.
References
i. O’Rourke, Ronald. “China Naval Modernization: Implications for U.S. Navy Capabilities—Background and Issues for Congress.” December 1, 2022.
ii. O’Rourke, Ronald. “Navy DDG-51 and DDG-1000 Destroyer Programs: Background and Issues for Congress.” 2011. Pages 6, 12, and 25. Average Costs for New Flight IIA Destroyers based on averaging multi-year procurement of DDGs 114-116, coming to $1,847 Million per ship.
iii. O’Rourke, Ronald. “Navy DDG-51 and DDG-1000 Destroyer Programs: Background and Issues for Congress.” 2022. Page 25. Table A-1. Per ship cost determined based on “Estimated Combined Procurement Cost of DDGs 1000, 1001, and 1002” in millions as shown in annual Navy budget submissions, using the FY23 Budget submission dividing the three ships’ cost by three.
iv. O’Rourke, Ronald. “Navy LPD-17 Flight II and LHA Amphibious Ship Programs: Background and Issues for Congress”. 2022. Pages 1 and 6. AND https://www.navy.mil/Resources/Fact-Files/Display-FactFiles/Article/2169795/aircraft-carriers-cvn/
v. O’Rourke, Ronald. “Navy Virginia (SSN-774) Class Attack Submarine Procurement: Background and Issues for Congress” 2021. https://www.documentcloud.org/documents/20971801-rl32418-12 Page 9.
vi. O’Rourke, Ronald. “Navy Large Unmanned Surface and Undersea Vehicles: Background and Issues for Congress.” 2022. Page 9.
vii. Congressional Budget Office. “An Analysis of the Navy’s Fiscal Year 2023 Shipbuilding Plan”. 2022. https://www.cbo.gov/publication/58447 Table 7, “Average Costs per Ship Over the 2023–2052 Period for Flight III DDG”.
viii. Ibid, for FFG-62 Frigates.
ix. O’Rourke, Ronald. “Navy Constellation (FFG-62) Class Frigate Program: Background and Issues for Congress”. 2021. Congressional Research Service. https://sgp.fas.org/crs/weapons/R44972.pdf
x. CBO. Navy FY23 Shipbuilding Plan Analysis. Table 7. “Average Costs” DDG(X).
xi. Ibid. “Average Costs”. LPD(X), LHA-6, CVN-78.
xii. O’Rourke, Ronald. “Navy Virginia (SSN-774) Class Attack Submarine Procurement: Background and Issues for Congress” 2021. https://www.documentcloud.org/documents/20971801-rl32418-12 Page 9.
xiii. O’Rourke, Ronald. “Navy Large Unmanned Surface and Undersea Vehicles: Background and Issues for Congress.” 2022. Page 9.
xiv. O’Rourke, Ronald. “Navy DDG(X) Next-Generation Destroyer Program: Background and Issues for Congress” 2022. Page 2.
Featured Image: The guided missile destroyers USS Mustin (DDG 89), foreground, and USS Curtis Wilbur (DDG 54) steam through the Philippine Sea during a replenishment at sea Sept. 18, 2013. (U.S. Navy photo by Mass Communication Specialist 3rd Class Paul Kelly/Released)