Read Part 1 on defining distributed maritime operations.
Read Part 2 on anti-ship firepower and U.S. shortfalls.
Read Part 3 on assembling massed fires and modern fleet tactics.
Read Part 4 on weapons depletion and last-ditch salvo dynamics.
By Dmitry Filipoff
Introduction
There is more to the lethality of a volume of fire than sheer numbers. Missile salvos can take on different patterns, both in how the missiles are arranged within a single salvo, and how multiple salvos can be arranged together into a combined volume of fire. These patterns reflect how the aspects of concentration and distribution apply to the weapons themselves, and how these configurations apply within salvos and between salvos. Different patterns will affect how a volume of fire takes shape and can multiply the threat it poses. Commanders and autonomous missiles can leverage these patterns to increase tactical advantage by changing how salvos are maneuvered throughout key elements of the fight. These patterns have considerable tactical implications for defending against missiles and maximizing offensive volume of fire.
Stream versus Saturation
It is infeasible for a warship to instantly fire a large volume of missiles all at once. While warships can certainly fire missiles rapidly, their rate of fire is typically limited to only a few missiles at a time from the whole of their launch cells.1 Because the entirety of a salvo cannot be fired at once, salvos often default to a stream pattern, where a long, vertical column of missiles travels toward a target (Figure 1). Each missile in the column is slightly further away from the target than the missile ahead of it, because each missile was fired slightly later than the missile before it.
Figure 1. Click to expand. A warship launches a salvo in a stream pattern. (Author graphic via Nebulous Fleet Command)
This typical salvo pattern has several disadvantages, such as how an attacking stream salvo can allow the defender to more easily defeat the missiles in detail. If the streaming missiles are flying closely enough along the same flight path, destroying missiles at the head of the stream can disrupt missiles further behind as they may have to fly through exploding shrapnel and debris. A stream salvo can also minimize the maneuvering and targeting readjustments needed to apply warship defenses that are more directionally limited, including mounted defenses such as laser dazzlers, rolling airframe missile launchers, and close-in weapon systems (Figure 2).
Figure 2. Click to expand. A close-in weapon system engages multiple missiles of a stream salvo approaching on a single axis. (Author graphic via Nebulous Fleet Command)
Alternatively, a saturation pattern has greater tactical advantages. Instead of flying in a staggered sequence or vertical column, the missiles are traveling abreast of one another in a wide horizontal row. This salvo pattern poses a broad front of concentrated firepower compared to the long and narrow front of a stream salvo, where a saturation salvo takes the form of a multi-axial attack instead of the stream’s single axis. Once a saturation salvo crosses over the horizon, all of the missiles aim to be at a similar distance from the target warship, intensifying the challenges of defense. Directional defenses will need to traverse more angles to acquire new targets, and the attacking missiles run a lesser risk of having to fly through the exploding debris fields of their destroyed colleagues (Figure 3).
Figure 3. Click to expand. A close-in weapon system engages a saturation salvo’s missiles across multiple axes. (Author graphic via Nebulous Fleet Command)
Platforms that feature small magazines and larger numbers, such as aircraft, truck launchers, and small missile ships can more readily assemble into firing formations that generate saturation patterns from the onset. But the feasibility factors that bottleneck a warship’s rate of fire make a saturation pattern more unnatural for a warship to launch than a stream pattern, where warships can only launch large salvos in streams. The missiles will have to be maneuvered into a saturation pattern after the salvo is fired. Ideally this will be facilitated by networking and autonomy on the part of the missiles, rather than a complex firing scheme on the part of the launch platform. Modern anti-ship missiles will be able to be programmed to self-organize into a saturation pattern after they are launched from a warship, or outside retargeting and in-flight updates could provide similar instructions.2 By maneuvering weapons after they are launched, these capabilities serve the critical function of helping a salvo’s missiles maximize the overlap of impact timing regardless of the launch platform’s rate of fire.
In salvo combat, if a missile is not able to hit the target because it was shot down by defenses, the next best thing is that its destruction can buy a sliver of time for another missile in the salvo to have a slightly better chance of striking. This dynamic can continue throughout an engagement, where through their destruction, missiles are buying small consecutive improvements in striking opportunity for other missiles. The way impact timing is distributed across a salvo’s missiles will affect how much opportunity the destroyed weapons purchase for the survivors. A majority of a salvo’s missiles will likely be destroyed in the process of ensuring that only a handful have a real chance of striking the killing blows.
Stream patterns stretch the volume of fire thin with respect to the timing of impact. Each missile in the salvo would impact the target at a slightly later time than the missile ahead of it, where the distribution of impact times across a stream salvo is mainly limited to being a function of the launching warship’s rate of fire. If defenses are robust enough, a defender can even keep a stream salvo at a fixed distance away from the target until the salvo is fully destroyed. The ability for stream salvo missiles to buy time for one another is diminished by how destroying the missile at the head of the pack slightly rolls back the clock.
What a saturation pattern offers is a salvo that is always closing the distance regardless of the extent of attrition. Even if missiles are being destroyed, the minimum time to impact is steadily winding down. The volume of simultaneous defensive effects required to keep the whole of a saturation salvo at a fixed distance away from a target warship is far higher than that of a stream salvo, because it would require destroying the entirety of the saturation salvo simultaneously.
A saturation salvo epitomizes the principle of concentrating effects, where all missiles in the salvo are angling to strike the target at the same time, and bring the full weight of the entire volume of fire to bear at once. Saturation salvos improve efficiency by maximizing concentration, and can reduce the number of offensive weapons required to overwhelm warship defenses.
Because defensive missiles typically have much less range and flight times than long-range anti-ship missiles, they have far less opportunity to be maneuvered into saturation patterns, especially when they must strike incoming missiles that are only miles or seconds away from impact. Saturation patterns can chiefly be a feature of attacking salvos, while a warship’s defending salvos are more likely to be relegated to stream patterns. This forms a critical asymmetry in the offensive-defensive balance and confers significant advantage to the attacker in naval salvo warfare.
Salvo Patterns and Tactical Information
One of the most critical considerations for preserving missile inventory and preventing wasteful fires is guarding against deception and maintaining quality targeting information while salvos are traveling toward distant targets. Salvo patterns heavily influence the tactics of missile search and deception, especially given how capable modern seekers have become.
Anti-ship missiles are difficult to evade and deceive when their onboard seekers feature a robust combination of sensor modes including infrared, electro-optical, active, passive, and others. These combined sensors are meant to work together to maximize their strengths while covering each other’s blindspots. They aim to simplify the challenge of terminal search while negating softkill capability. A passive radar receiver can often detect a target or radiating decoy at longer range than an electro-optical sensor, but the latter is much harder to deceive when the contact enters within visual range.3 At that range missiles will be especially challenging to deceive, where they are close enough to visually verify a target’s authenticity. And once they make their final approach, the missiles’ targeting logic can employ aimpoint selection capability, where they select the most lucrative impact points on a ship to maximize destructive potential, such as hitting a ship directly in its missile magazines.4 Aimpoint selection capability makes effective damage control a dubious proposition and helps ensure that only a single well-placed hit is enough to destroy a target, reducing the volume of fire necessary to inflict sufficient striking power.
These electro-optical and infrared sensors are major force multipliers by making it much easier for missiles to ignore the short-ranged warship-launched decoys that form a major portion of a warship’s softkill defenses. Even if these decoys pull a missile away from a ship at the last moment, an intelligent missile would know to circle back for another pass, where the decoy only buys the warship more time to shoot down the threat. Effective softkill deception against intelligent missiles therefore needs to occur at a distance that goes well beyond the horizon. Otherwise deception measures that occur within the horizon view of a warship will struggle to have an effect against missiles that can literally see the warship.
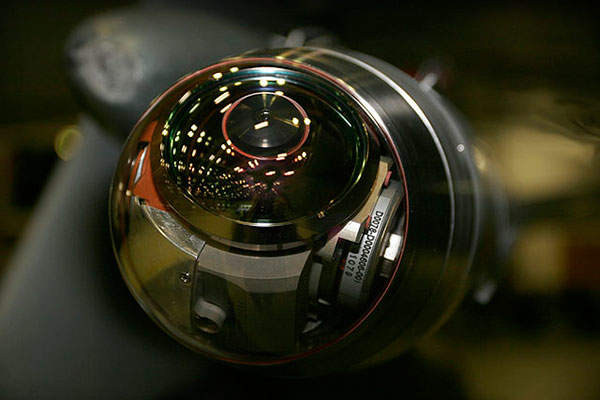
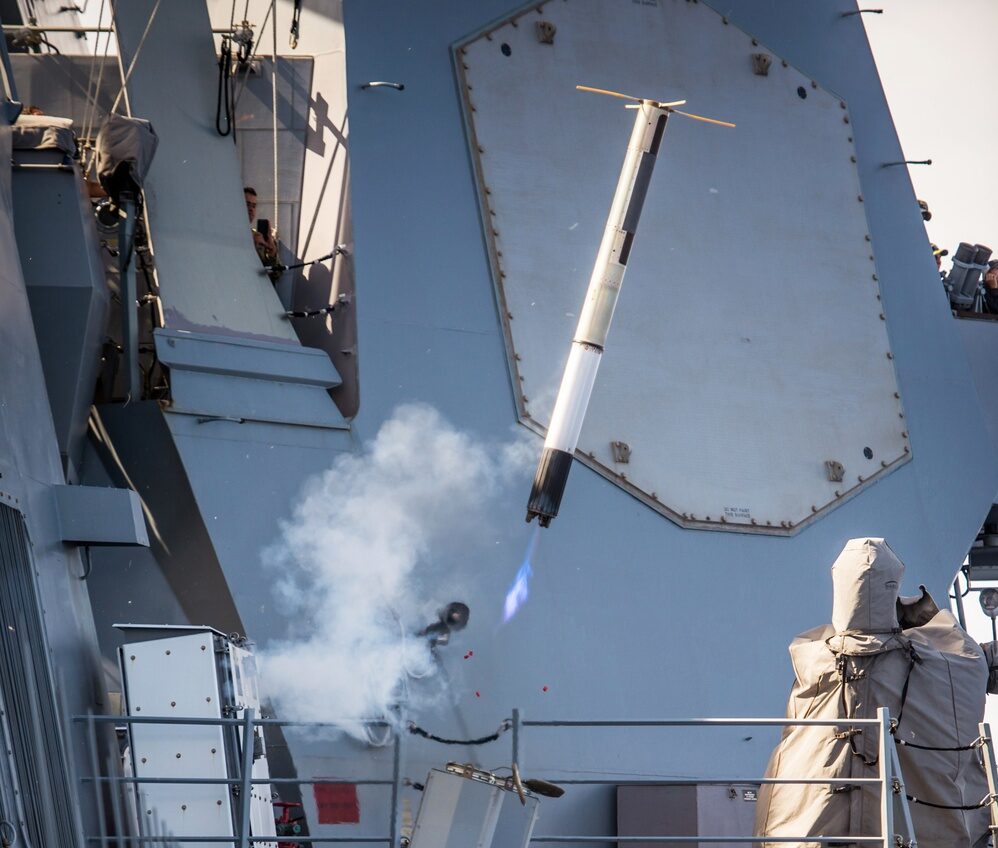
Successfully deceiving these anti-ship missiles may be less likely to take the form of getting them to strike false targets. It may instead become more a matter of keeping them at arm’s length and pulling them in directions away from friendly forces until they waste enough time and fuel that they fall from the sky. But most of a typical warship’s decoy capability is very short-ranged, and warships are extremely limited in their ability to deploy decoys tens of miles away from the ship. They may have to rely on other platforms such as aviation to deploy decoys at a tactically meaningful distance away from the warship.
Once a salvo is fired against a warship, an area of uncertainty grows around the target, where the warship may have moved from its original position at the time of launch, and where decoys may be deployed within this area of uncertainty. This area of uncertainty remains relatively small for the speediest weapons and missiles with short times-to-target. But for long-range and subsonic weapons, this area can grow to include thousands of square miles.5 If the area of a missile seeker’s coverage can overlap most of the area of uncertainty, then the problem of terminal homing is somewhat simplified. But if the area of uncertainty exceeds seeker coverage, then missiles may need to rely more on their own search capabilities to find and discriminate contacts for attack in the final phases.
Saturation patterns maximize the ability of a salvo to search and find a target. A saturation pattern spreads missile seekers across a wide front, allowing each seeker to search a given axis (Figure 5). If a seeker acquires a target, in-flight networking and autonomy between missiles can allow them to converge on a specific contact. A stream salvo by comparison makes for a highly redundant search pattern by concentrating seekers along a single axis, which is hardly ideal for searching across an area of uncertainty (Figure 4).
Figure 4. Click to expand. The seekers of a stream salvo pattern search along a narrow axis. (Author graphic via Nebulous Fleet Command)
Figure 5. Click to expand. The seekers of a saturation salvo search across multiple axes. (Author graphic via Nebulous Fleet Command)
Missiles can be drawn to contacts that turn out to be decoys, which may need to be discriminated by much shorter-ranged seeker modes than radar, such as electro-optical or infrared sensors. The need for missiles to close the distance to more rigorously investigate and verify contacts can threaten the cohesion and range of the salvo. Relying on only a few missiles at the head of a stream to do most of the searching on behalf of the salvo runs a greater risk of having the whole salvo being led astray by false contacts, which will come at a significant cost to fuel, range, and time. Advanced networking and autonomy may do little to alleviate the inherent tunnel vision of a stream salvo, where the whole of the salvo can be made to suffer penalties if only the leading missiles are deceived. If the missiles lack the programming and networking to work together, a stream salvo encountering a decoy could fragment and lose its cohesion as some missiles take the bait and others do not.
In a primitive stream salvo, the pattern of searching for a target and attacking a target remains virtually the same, compared to the more dynamic expansion and contraction of a saturation salvo that widens while searching for a target and then converging on it. A saturation salvo is much better able to withstand the disruption decoys can inflict against the coherence of the volume of fire. When the salvo is searching across a wide front, a single weapon could investigate a contact and make sure to only cue the rest of the salvo to converge on the contact after it has been verified. This helps a saturation salvo reduce the cost of deception to a single weapon or a handful of weapons being led astray at a time, rather than larger segments of the salvo like a stream pattern. However, if the deception is effective enough to get networked missiles to cue convergence, then a saturation salvo that is made to repeatedly expand and contract as it converges on false contacts and then renews its search is a salvo that will be quickly running down its mileage.
Stream salvos may offer some informational advantages when it comes to battle damage assessment and assessing the effectiveness of an attack. Missiles later in the stream could use their sensors to perceive that the target ahead has been destroyed and communicate fresh battle damage assessment information to the network. Or they could communicate that the vast majority of the missiles ahead them have been destroyed by defenses and strongly suggest that a salvo is on the verge of being defeated. In either case, missiles could deliver especially critical and time-sensitive intelligence on the effectiveness of attacks and defenses, assuming they are able to deliver such information through a network in those contexts. A saturation salvo that simply maintains several weapons to the rear of the main wave of attacking missiles could deliver similar information.
If the targeting and search capabilities of salvos are capable enough, they can lower the threshold of information required to precipitate a strike and speed the decision cycle. If the missiles are capable enough to sort out contacts and even decide their own distribution of fire across a target naval formation, then commanders can launch on less information knowing the missiles themselves can reliably sort out critical details. If an adversary is presenting a mass of cluttered signatures that makes target discrimination difficult from afar, saturation salvos could be fired into the mass in a bid to earn positive identification themselves and function as one-way scouts. Modern seekers that can visually identify a target based on robust onboard databases of warship designs should be capable enough to differentiate most warships from civilian vessels and minimize the ability of navies to use commercial traffic as human shields.
With respect to the vulnerability of the launch platform, a stream salvo can more easily betray the position of its launching warship by providing a clearly defined line of bearing back toward the vicinity of the platform. A warship under attack from a stream salvo could fire its offensive weaponry down this line of bearing in a last-ditch salvo and have a greater chance of striking back. Nonlinear flight paths and saturation patterns can help mitigate this risk through multi-axis attacks that can manipulate perceptions of where an attack originated.
But nonlinear attacks and saturation patterns incur penalties in range and fuel economy. Stream salvos will suffer less penalties than saturation salvos in this regard because it is more fuel efficient to maneuver a salvo across waypoints when maintaining a stream pattern. By comparison, saturation salvos will suffer a greater cost in fuel given how some missiles will have to cover more distance than others to preserve the abreast formation while traveling across waypoints. It may be more preferable to confine a saturation pattern to the terminal phase of attack rather than the cruise phase of missile flight, where a stream salvo only expands into a saturation profile just before breaking over the target’s horizon.
Salvo patterns can therefore be flexed during flight to emphasize search, fuel economy, or lethality depending on what is more applicable at various points in the engagement. The need for maximizing range and fuel may compete with the need to search and withstand deception, where these latter factors encourage a saturation pattern. If enough outside retargeting support can confidently convey information to a salvo during flight, then it can minimize the amount of fuel the salvo would have to expend in a broader search pattern. This can also improve the survivability of the salvo and improve its element of surprise, where a saturation pattern engaged in search could provide more early warning to an adversary by posing a radiating wall of missile seekers. Even emphasizing passive detection can reduce the element of surprise, since missiles may have to leave sea-skimming altitudes to broaden the reach of their sensors. Outside retargeting support is helpful toward improving the range and survivability for missile salvos on their way to the target by allowing them to maintain low-altitude stream patterns, and reduces the need for saturation patterns to only the final moments of attack.
A salvo of Soviet P-500 Bazalt anti-ship missiles (NATO reporting name: SS-N-12 Sandbox) is fired by a Slava-class cruiser against a U.S. Cold War-era surface action group. Demonstrated intelligent missile swarming behaviors include self-organization from stream pattern into saturation pattern, single high-altitude missile searching on behalf of larger sea-skimming salvo, target prioritization for distribution of fire, and weaving flight profiles in terminal attack phase. Blue trails mark offensive missiles, pink trails mark defensive missiles. (Work-in-progress developer video of forthcoming naval wargame, Sea Power: Naval Combat in the Missile Age.)
Patterns of Combining Fires
Saturation and stream patterns go beyond describing individual salvos, where they can also describe the broader aggregated salvo as a whole. Depending on how contributing fires are being amassed from distributed forces, the aggregated salvo itself may take on an overall stream or saturation profile, or some mixture of the two. The overall profile of an aggregated salvo may feature an amalgamation of waypointing and salvo patterns that generate especially complex threat presentation as a shapeshifting volume of fire closes in on a target (Figure 6).
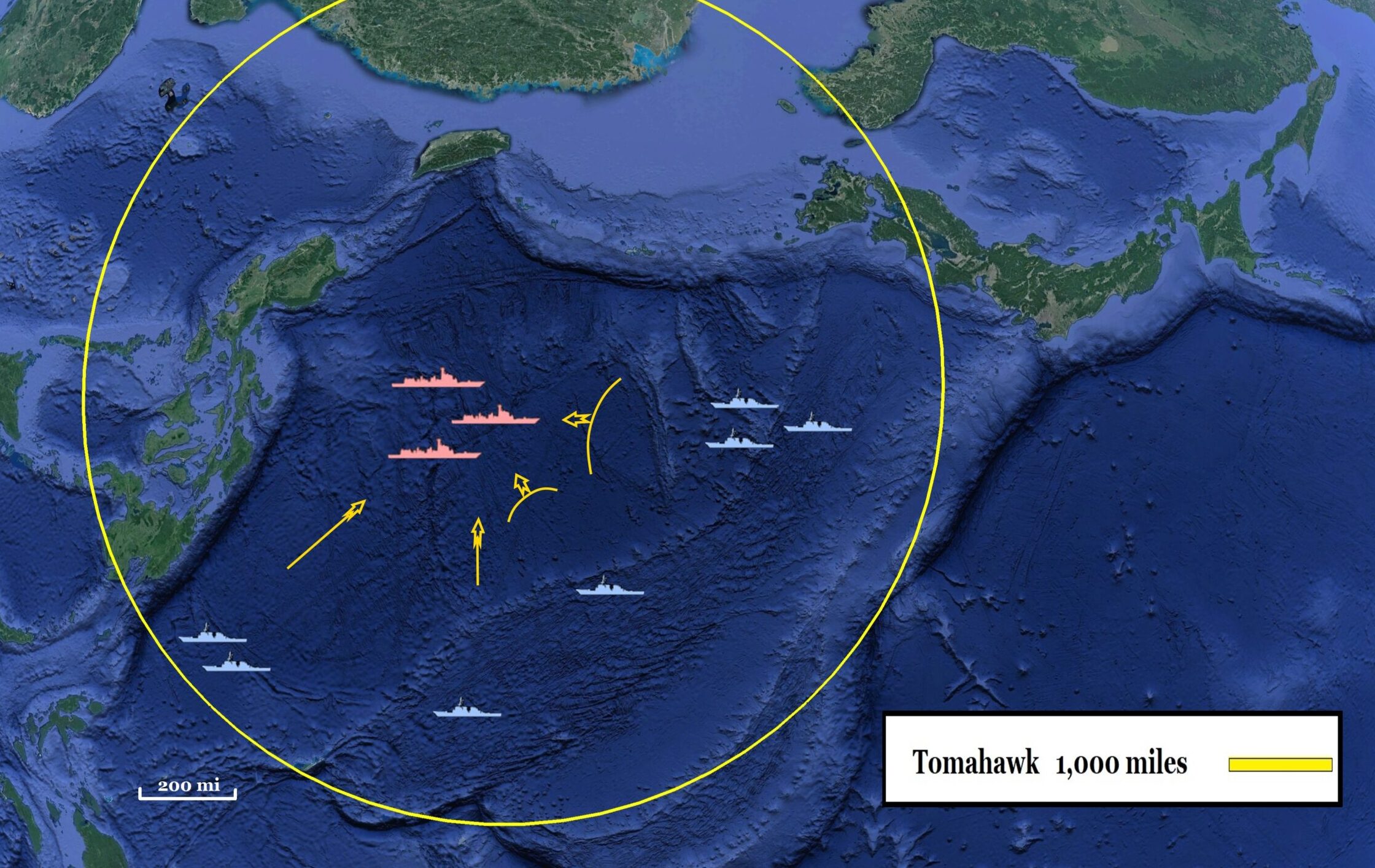
It is easier to combine with stream salvos than saturation salvos. Because not all missiles in a stream salvo will hit the target at the same time, there is slightly more opportunity to overlap with the salvo, which can measure in the tens of seconds. A saturation salvo will pose greater challenges for effective aggregation because the salvo is already attempting to position all its missiles to strike the target at the same time. Outside salvos attempting to combine with saturation salvos will have to be very closely aligned in timing because of the minimum of opportunity for overlap.
In-flight retargeting and programming can play a critical role in ensuring aggregation can maximize opportunity for saturation. Multiple contributing salvos can approach a target as streams and then travel waypoints in a holding pattern beyond the target’s horizon until more contributing fires arrive. Once the final attack is initiated, the contributing fires switch to saturation patterns and converge on the target. The efficiency of the stream pattern buys more time to grow the volume of fire, and the lethality of the saturation pattern is reserved for the final approach.
As various contributing fires approach a target, the defenders may prioritize the destruction of specific salvos based on their patterns. Defenders may prioritize saturation patterns especially, believing them to be the greater threat. The more complex the flight profile and missile behavior, the more an adversary may assume that a set of contributing fires consists of more capable missiles, and prioritize those salvos for interception by its defensive airpower and other means.
Salvo patterns can be flexed to manipulate adversary threat perceptions and potentially open gaps in defenses. By flexing a combination of salvo patterns and waypoints, a set of contributing fires could expand into a saturation profile to draw adversary airpower away from a target and open opportunities for other salvos to make the strike. And as a salvo comes under attack from airpower, it can shift its flight profile as it senses radar illumination and notices that friendly missiles are disappearing from the local network. By shifting flight profiles while under aerial attack, a missile salvo can make defense more challenging and buy time for the overall strike. Primitive anti-ship missiles by comparison may hardly change their flight behavior when under attack or radar illumination, simplifying the defender’s challenge.
Salvo Patterns: A Forthcoming U.S. Advantage?
The ability to leverage the tactical advantages of salvo patterns may be one of the key advantages the U.S. will have over China by fielding the anti-ship capable variants of the Tomahawk missile, assuming China does not develop similar weapons. The Tomahawk missile’s especially long range gives it great flexibility for maneuvering through various patterns and along many waypoints. Greater range also improves the missile’s ability to recover from deception by false contacts and extend its search for real targets. These capabilities are magnified by another dimension of salvo patterns, that of sea-skimming versus high-diving attacks.
Anti-ship ballistic missiles can take on saturation patterns by virtue of being launched by multiple platforms with shallow magazines, such as truck launchers. But despite having similar range as Tomahawk, anti-ship ballistic missiles are heavily disadvantaged when it comes to reconfiguring their salvo patterns in real time. The fixed nature of a ballistic trajectory strongly constrains the ability of these weapons to alter the disposition of their salvos while in flight, and the steep high-diving nature of their final approach constrains the scope of ocean their onboard seekers can search across.6 A ballistic missile on its terminal descent cannot decline a false contact and then default back to a wider search pattern as easily as a cruise missile. A ballistic missile locked into its terminal descent is only moments away from hitting the ocean regardless of whether its targeting information is viable or not, whereas a cruise missile has more margin for error. By their nature, ballistic missile attacks attempt to minimize the area of uncertainty around a target not so much by coordinating search across a salvo’s seekers, but more by having tremendously high speed that helps preserve the viability of the original targeting information given at launch.
The differences in terminal search and attack patterns between ballistic missiles and cruise missiles is somewhat similar to that of the attack profiles of WWII dive bombers and torpedo bombers, respectively. The dive bomber, like a ballistic missile, makes its final approach from a steep angle at higher altitude, exposing itself to a broader array of sensors and defensive firepower, while having relatively little leeway to shift to new targets midway through its high-speed dive. The torpedo bomber by comparison is usually traveling more slowly, but its flight profile is at a flatter angle that affords it much more maneuverability, even in the terminal attack phase. This flatter flight profile offers a broader scope of opportunity to investigate contacts, recover from deception, and shift targets, while giving the platform more options in when it begins its terminal approach.
A sea-skimming cruise missile is therefore better able to employ a wider search pattern across the area of uncertainty around a target than a weapon locked into a high-diving flight profile. While the visibility of sea-skimmers is more deeply affected by horizon limits than high-divers, a high-diving platform or missile may struggle to radically reorient itself toward a new contact during its dive, and the smaller size of the seekers used by missiles can limit how much those weapons can leverage the broader visibility for search. However, sea-skimming attackers may have to break through successive layers of defending warships and aircraft before they can threaten a priority target in the interior of a formation. In exchange for some disadvantages, high-diving attackers can threaten those priority targets directly.
Conclusion
Sharpening the intelligent swarming behaviors of anti-ship missiles will be a key area of naval competition, one with significant potential for building offensive advantage. These capabilities should be expected to proliferate and magnify missile threats. Navies should take care to assess the programming and autonomous targeting logic of their salvos to consider how this may make their striking power concentrated or stretched thin during an attack. When warship salvos have little in the way of effective networking or autonomy, they default to more primitive stream salvo patterns and suffer major disadvantages. They become more susceptible to deception, struggle with long-range search, and raise the cost of attack.
As a distributed force masses its fires, it will attempt to maximize the saturation effect. In those final phases of attack, the greatest offensive advantage will be gained when saturation patterns characterize how salvos are arranged as they converge on a target. What these salvo patterns make clear is that in the missile age, the weapons themselves have become a chief maneuver element.
Part 6 will focus on the strengths and weaknesses of platform types in distributed warfighting.
Dmitry Filipoff is CIMSEC’s Director of Online Content and Community Manager of its naval professional society, the Flotilla. He is the author of the “How the Fleet Forgot to Fight” series and coauthor of “Learning to Win: Using Operational Innovation to Regain the Advantage at Sea against China.” Contact him at [email protected].
References
1. Factors that bottleneck a warship’s rate of fire from vertical launch systems include exhaust gas limits and safety risks of launching numerous missiles in very close proximity to one another. The minimal unit of a MK41 vertical launch system takes the form of eight-cell module, with each module sharing a common exhaust gas system.
For module and exhaust gas design of MK 41 VLS, see:
Eric Fiore, “A Promising Future for US Navy Vertical Launch Systems,” DISIAC Journal, Volume 1, Number 2,, pg. 35, Fall 2014, https://www.dsiac.org/wp-content/uploads/2020/05/dsiac-journal-fall-web-1.pdf.
2. John Keller, “Lockheed Martin to build six more LRASM anti-ship missiles with GPS/INS, infrared, and radar-homing sensors,” Military and Aerospace, March 23, 2022, https://www.militaryaerospace.com/sensors/article/14248345/multimode-sensors-antiship-missiles.
3. Wayne P. Hughes and Robert Girrier, Fleet Tactics And Naval Operations, Third Edition, pg. 188, U.S. Naval Institute Press, 2018.
4. For aimpoint selection capability, see:
“JSM: Joint Strike Missile,” Kongsberg, https://www.aerocontact.com/public/img/aviaexpo/produits/catalogues/92/Brochure-Joint-Strike-Missile.pdf.
5. Jeffrey R. Cares and Anthony Cowden, “Fighting the Fleet: Operational Art and Modern Fleet Combat,” U.S. Naval Institute Press, pg. 30-42, 2021.
6. Gerry Doyle and Blake Herzinger, Carrier Killer: China’s Anti-Ship Ballistic Missiles and Theater of Operations in the early 21st Century, Helion & Company, pg. 42, 2022.
Featured Image: US joint forces conducted a sinking exercise on the decommissioned guided missile frigate ex-USS Ingraham in the Hawaiian Islands Operating Area, 15 August 2021. (US Navy photo MCS David Mora Jr)