Read Part One here.
By Tyler Cross
Strategy and Security – Canada’s Perspective
Canada, much like Russia, is heavily invested in the Arctic. Ranking second in Arctic interests, it also ranks second in land holdings with 1.2 million square miles found above the Arctic Circle. Canada’s northern borders have also long been secured by the vast expanses of Arctic ice, but they may find their Arctic holdings precariously exposed if ice sheets become non-existent.
The Northwest Passage dispute involving Canada is set to come to the forefront of security concerns as it becomes increasingly navigable. This dispute, however contentious, is unlikely to seriously threaten regional stability. At the same time, a solution does not appear to be in the making. The Canadians have lesser disputes over parts of the Lincoln Sea and Hans Island with Denmark and parts of the Beaufort Sea with the U.S.1 But all disputes are with staunch NATO allies, and they are poised to find diplomatic solutions.
There exists a dichotomy of Canadian strategy in the Arctic. Diplomatically, Canada tends to take a “lean back” approach, but at the same time there is a consistent focus on the Arctic. Of all 28 NATO countries, Canadians are the least excited to see an allied military presence in the Arctic. Multi-national military presence there, they feel, will undermine their near hegemony in large portions of the high latitudes. Anti-military cultural bias is relatively higher in Canada, and a significant portion of the populace ardently supports indigenous societies who call the Arctic home. The Canadians prefer decisions to be made by the diplomatic and intergovernmental Arctic Council as opposed to the more military-oriented policies of NATO.2 In sum, there is a general aversion to military development in the Arctic.
But ice cap depletion may bring a change of heart on High North militarization. While much of Canadian Arctic policy is characterized by a “lean back” strategy, there has been a bit of a pivot to the North in recent years. The Canadian military has developed its operational capacity in cold, remote regions. Recently, the Canadian military has purchased a new icebreaker to complement its six working ships, while creating a winter fighting school and a deepwater port in Baffin Bay. Likewise, former Canadian Chief of Defense General Walter Natynczyk has an appreciation for Arctic defense and began increased allotments to strategic security there.
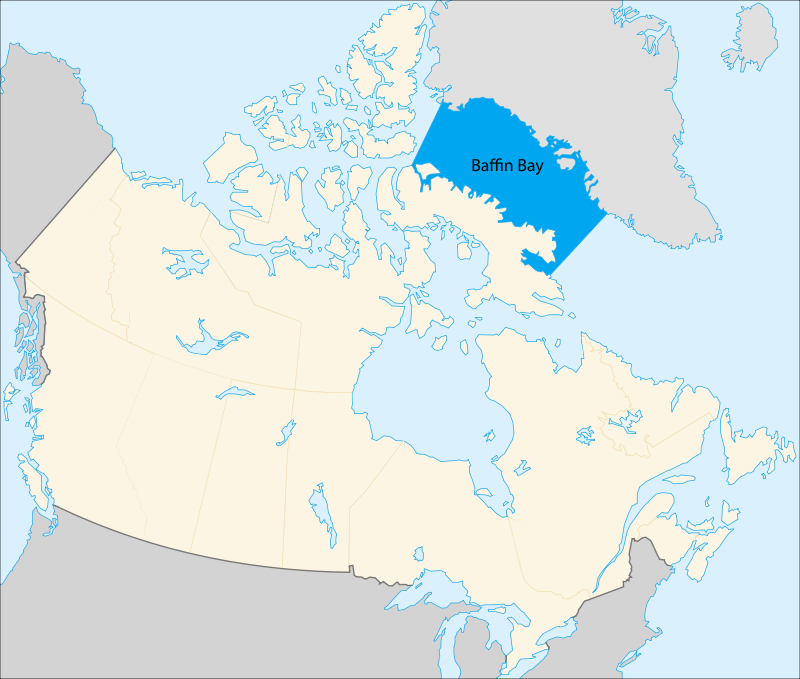
The Canadians are willing to protect assets that they see as rightfully theirs, but are inclined to pursue multi-nation diplomacy at the same time.3 The dichotomy appears a bit contradictory, and they would do well to continue Arctic operations, even if they are limited. This would help rebut Russian advances and strengthen general security. The Canadians are strong NATO members and have participated in coalition efforts worldwide, making them a vital cog in mutual defense.
Strategy and Security – European NATO States
Norway favors the most proactive Arctic defense policy of all NATO nations. They see Russia as particularly threatening, and recent history has only validated their claims. Norwegian security is driven by defense against Russia, as they feel the shadow of the resurgent giant looming over their relatively small population of five million. The Norwegian island of Svalbard, according to former NATO supreme allied commander Admiral James Stavridis, “constitutes a significant thorn in the side of Russian ambitions in the region.” Fisheries teem off the icy island’s coast, and are of great value to the Norwegians. Defense of the island and its nearby fish stocks are paramount and will be important in future defense strategy. Hydrocarbons are also of concern, and will become increasingly important as easily extracted oil is depleted on the mainland.4
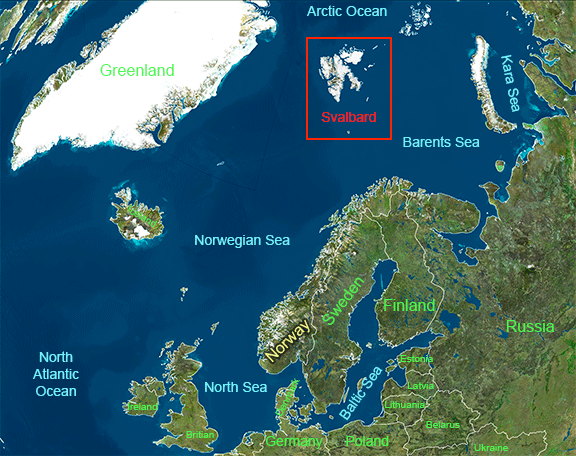
Of the NATO members, Norway has the most active military in the Arctic. They see the region as the alliance’s unguarded flank, and constantly prompt other member states to be well-informed and combat ready.5
In 2008 Russia resumed surface patrols in the waters surrounding Svalbard. The Norwegians found this deeply concerning, and it has only prompted further active preparation and defense readiness. A microcosm of the tensions played out when in 2005, one Russian fishing vessel, the Elektron, encroached on exclusive fishing zones off the coast of Svalbard. It was chased by a Norwegian Coast Guard ship. Tense days ensued, but the dispute was eventually resolved diplomatically.6 The Elektron incident was indicative of future fishery confrontations and tensions between the two Arctic states as a whole. Luckily, the diplomatic solution resolved the affair peacefully, but one must also remember that Russia was not as powerful or assertive on the world stage in 2005. While still unlikely, the chances of more confrontations are higher today than they were before. Norway, feeling rather threatened by Russia, has continued its “lean forward” policy in the years since.
Iceland was viewed as an unsinkable aircraft carrier during the Cold War. It would again be stuck between the United States and Russia in a Cold War 2.0 in the Arctic. Iceland desires the waters to their north to be a zone of cooperation. Ideally for them, Reykjavik would become a stop and central location to a North Atlantic trade highway. With oil to be found within their 200 mile exclusive economic zone, there is much economic growth potential.7 Iceland may look to distance itself militarily from the United States if a free economic zone is achieved, or if the island’s leaders fear they could become ensconced in another Cold War. It is also likely that the American military presence will reappear in Keflavik, particularly in response to Arctic tensions. If Icelanders feel threatened, they look to the U.S. Navy to assuage concerns. Iceland was a pivotal halfway point during the Second World War, and could serve as a vital way-station for the future. They are also close allies with the Danes, and this close alliance and increasing needs for mutual defense could bring the two states further into NATO and closer to the U.S.
Denmark is an Arctic state by virtue of Greenland and the Faroe Islands. Greenland has been an important location for NATO defense, especially because of the bases the Danes provide. In addition to its strategic importance, predominantly through early missile warning, Greenland has increased in importance lately with the discovery of natural gas deposits, and Denmark will look to aggressively map the Arctic floor in search of more resources while simultaneously increasing military presence. Their claims are likely to go all the way to the North Pole itself, and Copenhagen will look to back its claims with naval presence.8 In 2009, the Danes began establishing an Arctic military command.9
Iceland officially has no standing military, although its Coast Guard and Crisis Response Units are fully military in all but name. It is largely for this reason that the Danes have complete naval access to Icelandic ports. The two states also work as an alliance within the NATO alliance. Iceland acts as a strategic stopping point for Danish ships in transit between Greenland and Europe.
The United States should prioritize the Danes, as their provided NATO bases in Greenland are vital to Arctic defense. A relic of the Cold War, Thule Air Force Base, located on the island’s northwestern edge, was one of the most vital ICBM missile detection bases monitoring potential missile routes over the Arctic.
American Strategy – What To Do?
The NATO alliance helps defend the Arctic. Thule Air Base is a good example of cooperation in the Arctic, as it is home to approximately 400 Danes, 50 Greenlanders, three Canadians, and 140 Americans. Locked in ice nine months of the year, sea lanes are opened by a Canadian icebreaker. While extreme in location, being found 700 miles above the Arctic Circle, it is a good example of inter-alliance cooperation on top of the world.10 Activity in the Arctic is predicted to increase and could bring about similar bases in the future.
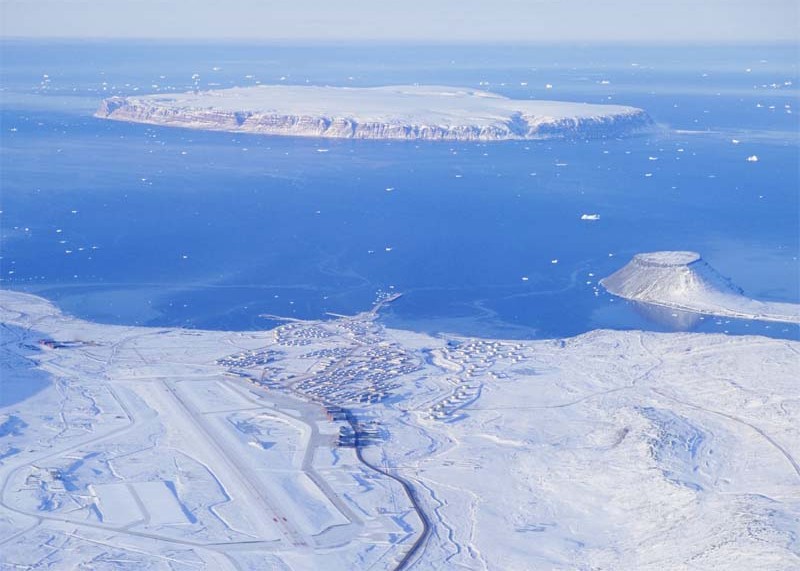
For the United States, this would likely begin in Alaska, where there has already been talk of further military development. But it will be important for planners to learn about the particular challenges and needs of Arctic militarization and prepare as traffic flows increase and the ice melts. The United States military will be unable to secure and protect all of the Arctic alone, and much of this will fall to NATO allies. Cooperation will be paramount in times to come.
The Russian Federation’s prioritization of the Arctic will be a primary driving factor in security strategy. Until 2009, the United States did not even have an articulated policy on the Arctic.11 But as the Arctic takes on new importance, American strategy will hopefully diversify and strengthen. Besides remote locations and harsh weather, the U.S. will face difficulty with a lack of inter-agency doctrine and cooperation in the Arctic. Plus the Arctic takes on new meaning with North Korea’s development of ICBMs that can reach the United States, where missiles have to travel through and over the Arctic in order to reach American soil.12 The U.S. will also have to consider both fighting and search and rescue forces, which will lack resourcing and enjoy limited interest from policy-makers.
But nonetheless, progress is being made. The Department of the Navy, including the Coast Guard and Marine Corps, have created a joint cooperative strategy that recognizes the opening of sea lanes in the Arctic. Their strategy recognizes new challenges. The Navy’s currently stated goal in the Arctic is “to foster and sustain cooperative relationships with other Arctic nations and, within the joint, interagency, international, and academic communities, to improve its understanding of the Arctic environment, enhance its ability to predict changes to it, and prevent or contain any regional instability, through the creation and maintenance of security at sea.”13 This is an encouraging start. There is a strong environmental focus, and understandably so. But Russian militarization in the High North will require the further development of security in this somewhat neglected region.
The United States needs to foster close allegiances and play a leadership role in NATO. In the summer of 2007, Russia explorers planted their flag on the underwater Lomonosov Ridge, deep in the Arctic and far beyond their exclusive economic zone. They claimed this underwater ridge for themselves.14 As if their militarization was not enough, the Russians are claiming international waters as their own, attempting to monopolize natural resources in the High North. It will take a concerted effort on the part of the NATO allies to rebuff the advances of the Russians, and deter them from claiming internationally recognized areas of open sea.
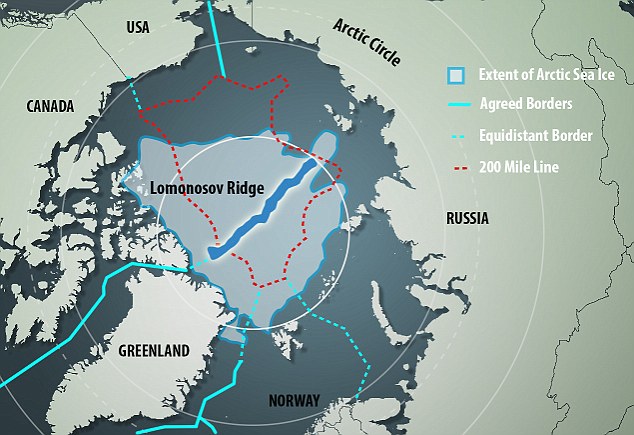
What is worse is that rules for free trade in the Arctic are meager. Moscow cannot be allowed to form hegemonic control of the Arctic and dictate terms retroactively. By mid-century, it is predicted that warm summers will see no polar ice coverage. If this happens, and Russia has an early and strong head start, freedom of navigation could be severely jeopardized. Plus, some of Russia’s Arctic stations are mobile.15 Control and mobility would provide a substantial advantage in a zone of conflict. By comparison, NATO stations are stagnant and sporadic. It will take a rejuvenated effort and concern for defense to appropriately meet this challenge.
The U.S. alone will be unable to counter Russian challenges, particularly north of the European theater. But help from NATO allies and close friends will act as a counterbalance. It will not take a large force to provide security in the Arctic, but a well-equipped and mobile one. Arctic exercises with active NATO members will be highly beneficial in identifying problems with joint warfighting maneuvers. Finland, Norway, Canada, and Denmark should be incorporated in these activities, and other allies should be invited. Swedish involvement would be ideal. It would also be wise to consider opening the military base in Keflavik, Iceland again. It was decommissioned as a military station just over a decade ago and is an active airport today. The station could help keep an eye on the growing Russian submarine fleet, and would greatly benefit both the Navy and Air Force to gather intelligence from this forward observation post. Additionally, its proximity to Reykjavik would make it a more tolerable post for servicemen when compared to other High North stations like remote Greenland.
In order to avoid security dilemmas, military exercises should be complemented by dialogue with Russia. One of the best avenues of discussion is via the Arctic Council, where Russia and the Arctic NATO states are members. Council meetings occur frequently, and include scientific, military, and geopolitical topics.16 Dialogue through the Arctic Council will be one way to soothe relations with Moscow in this region.
Break the Ice
Building icebreaker capability will be absolutely paramount to security and science in the Arctic. Icebreakers provide the ability to maneuver and supply remote bases. It is here that the United States is at its greatest disadvantage when compared to Russia. Currently the United States Coast Guard operates only one active icebreaker, the Polar Star. The other two icebreakers are inactive and at the end of their service life. And the Polar Star, many years removed from her christening, has seen 40 years of service and is set to retire in the early 2020s. By contrast, Russia operates 40 icebreakers, seven of which are nuclear powered. They are also building their new icebreaking nuclear powered flagship, the Arktika, slated for service before 2020.17
Russian nuclear-powered icebreaker Taymyr smashes through layers of ice just feet away from a group of adventurers in northwestern Russia. (Anton Panov/Oper_11 via Instagram)
The previous Commandant of the U.S. Coast Guard, Admiral Paul Zukunft, implored Congress to fund the building of new icebreakers. He found success, albeit somewhat limited. Zukunft asked for three heavy and two medium ships, and the first heavy icebreaker will be completed in 2023.18 This is an encouraging start, but the U.S. has a long way to go before it can compete with Russia or even smaller states in frozen seas. Finland has seven, Sweden seven, Canada six with another on the way, Denmark four, and China three with more in development.19 Congress will need to get serious about funding if it wants to compete in the Polar regions and enforce freedom of navigation in navigable ice flows. Luckily, the construction of each icebreaker pales in comparison to substantial Navy projects. The cost will be comparatively low, but the repercussions great.
The advantage of building icebreakers is that they facilitate freedom of navigation and trade in the Arctic. While the ice may be receding, northern shipping lanes will still be covered in ice for much of the year. Some lanes that have opened up remained locked in ice year-round, but the ice is thin enough for an icebreaker to pass through.
Ships looking to traverse the open ocean cannot rely on Russian help. As nice as cooperation will be, it is unlikely. When speaking at the Heritage Foundation, Admiral Zukunft said “I don’t think you’ll see tranquility about to break out.”20 Likewise, he openly discussed the idea of outfitting future icebreakers with cruise missiles. It suffices to say that it will take until 2023 for the next U.S. icebreaker to be completed, and Russia will complete two more by 2020, both possibly outfitted with cruise missiles of their own.21
Conclusion
The High North will almost certainly be a zone of competition. If Russia can take a hegemonic role there, it will lean on its military presence and the relative lack of international rules and norms for it to control the region. The Chinese are not far behind. American absence from the Arctic has weakened its stance with respect to great power competition and serves to upend the Navy’s stated mission of freedom of navigation. In a contentious Arctic, Russia will be unlikely to provide icebreaker coverage to international shipping and will try to claim resources and open sea. The United States Coast Guard must lead the way, and find close support from the other military branches.
The United States must remain a player in the Arctic Council and coordinate NATO defense in Arctic and near-Arctic regions. As long as the NATO alliance is strong, Russia will be deterred from exceedingly brash actions. With a strong presence, freedom of navigation will reign and peace can continue.
Tyler Cross recently completed a master’s degree in International Security at George Mason University. He will continue his career in international security cooperation.
Bibliography
Bender, Jeremy. “This is What Its Like to Live at The US’ Most Remote Air Base.” Business Insider. Last modified Nov. 24, 2014. http://www.businessinsider.com/what-its-like-to- serve-at-thule-air-base-2014-11.
Clark, Wesley. “Russia: Cold War 2.0?.” Lecture at the Michael V. Hayden Center for Intelligence, Policy, and International Security, Arlington, VA, March 5, 2018.
Dibb, Paul. “The Geopolitical Implications of Russia’s Invasion of Ukraine.” Strategic and Defense Studies Center, June, 2014.
Eckstein, Megan. “Zukunft: Changing Arctic Could Lead to Armed U.S. Icebreakers in Fleet.” USNI News. May 18, 2017, https://news.usni.org/2017/05/18/zukunft-changing-arctic- environment-could-lead-to-more-armed-icebreakers-in-future-fleet.
Hill, David D. “Force Projection, Strategic Agility, and the Big Meltdown.” Naval War College. May 18, 2001.
Kofman, Michael. “Russia’s Fifth Generation Sub Looms.” U.S. Naval Institute, Proceedings Magazine 143, no. 10 (Oct. 2017).
Roberts, Kari. “Jets, Flags, and a new Cold War? Demystifying Russia’s Arctic Intentions.” International Journal 65, no. 4 (2010): 957-976.
Rozman, Gilbert. Strategic Thinking about the Korean Nuclear Crisis Four Parties Caught between North Korea and the United States. New York: Palgrave Macmillan, 2011.
Slayton, David and Brigham, Lawson W. “Can the US and Russia Preserve Peace in the Arctic?” Investor’s Business Daily, May 13, 2015.
Spohr, Kristina. “The Scramble for the Arctic.” New Statesman 147 (March 9-March 15, 2018): 22-27.
Stavridis, James. Sea Power The History and Geopolitics of the World’s Oceans. New York: Random House, 2017.
Titley, David and St. John, Courtney. “Arctic Security Considerations and the US Navy’s Roadmap for the Arctic.” Naval War College Review 63, no. 2 (2010): 35-48.
Todorov, Andrey A. “The Russia-USA Legal Dispute Over the Straits of the Northern Sea Route and Similar Case of the Northwest Passage.” No. 29 (2017): 62-75.
“Zukunft to Congress: US Must be Serious About Icebreaker Acquisition,” U.S. Naval Institute News. Last modified May 18, 2017, https://news.usni.org/2018/04/17/zukunft-congress-u-s-must-serious-icebreaker-acquisition.
Zysk, Katarzyna. “Russia’s Arctic Strategy: AMBITONS AND CONSTRAINTS.” Joint Force Quarterly. 57 (2010): 103-110.
References
[1] Stavridis, pp. 344-345.
[2] Ibid., pp. 345-347.
[3] Ibid., 345-346.
[4] Ibid., p. 351.
[5] Ibid., pp. 351-352.
[6] Roberts, pp. 958, 968.
[7] Stavridis, pp. 349-350.
[8] Ibid., pp. 350-351.
[9] Zysk, p. 109.
[10] Jeremy Bender, “This is What Its Like to Live at The US’ Most Remote Air Base,” Business Insider, last modified Nov. 24, 2014, http://www.businessinsider.com/what-its-like-to-serve-at-thule-air-base-2014-11.
[11] Stavridis, p. 353.
[12] Ibid., pp. 353-356.
[13] David Titley and Courtney St. John, “Arctic Security Considerations and the US Navy’s Roadmap for the Arctic,” Naval War College Review 63, no. 2 (2010): pp. 42-43.
[14] Roberts, p. 958.
[15] David B. Hill, “Force Projection, Strategic Agility, and the Big Meltdown,” Naval War College, May 18, 2001, pp. 4-5.
[16] Stavridis, p. 338
[17] Ibid., p. 357. Some estimate Russian icebreaker capability is higher, as much as 44 in fact (source: Eckstein article)
[18] Eckstein.
[19] Stavridis, p. 357.
[20] “Zukunft to Congress: US Must be Serious About Icebreaker Acquisition,” U.S. Naval Institute News, last modified May 18, 2017, https://news.usni.org/2018/04/17/zukunft-congress-u-s-must-serious-icebreaker-acquisition.
[21] Eckstein.
Featured Image: Crewmen walk across the ice toward the US Coast Guard icebreaker USCGC POLAR STAR (WAGB 10). The POLAR STAR, first Coast Guard icebreaker to circumnavigate the Antarctic continent, has paused during its journey to give crewmen “ice-liberty.” (PA1 Ed Moreth, USCG)